the Creative Commons Attribution 4.0 License.
the Creative Commons Attribution 4.0 License.
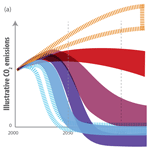
A perspective on the next generation of Earth system model scenarios: towards representative emission pathways (REPs)
Malte Meinshausen
Carl-Friedrich Schleussner
Kathleen Beyer
Greg Bodeker
Olivier Boucher
Josep G. Canadell
John S. Daniel
Aïda Diongue-Niang
Fatima Driouech
Erich Fischer
Piers Forster
Michael Grose
Gerrit Hansen
Zeke Hausfather
Tatiana Ilyina
Jarmo S. Kikstra
Joyce Kimutai
Andrew D. King
June-Yi Lee
Chris Lennard
Tabea Lissner
Alexander Nauels
Glen P. Peters
Anna Pirani
Gian-Kasper Plattner
Hans Pörtner
Joeri Rogelj
Maisa Rojas
Joyashree Roy
Bjørn H. Samset
Benjamin M. Sanderson
Roland Séférian
Sonia Seneviratne
Christopher J. Smith
Sophie Szopa
Adelle Thomas
Diana Urge-Vorsatz
Guus J. M. Velders
Tokuta Yokohata
Tilo Ziehn
Zebedee Nicholls
In every Intergovernmental Panel on Climate Change (IPCC) Assessment cycle, a multitude of scenarios are assessed, with different scope and emphasis throughout the various Working Group reports and special reports, as well as their respective chapters. Within the reports, the ambition is to integrate knowledge on possible climate futures across the Working Groups and scientific research domains based on a small set of “framing pathways” such as the so-called representative concentration pathways (RCPs) in the Fifth IPCC Assessment Report (AR5) and the shared socioeconomic pathway (SSP) scenarios in the Sixth Assessment Report (AR6). This perspective, initiated by discussions at the IPCC Bangkok workshop in April 2023 on the “Use of Scenarios in AR6 and Subsequent Assessments”, is intended to serve as one of the community contributions to highlight the needs for the next generation of framing pathways that is being advanced under the Coupled Model Intercomparison Project (CMIP) umbrella, which will influence or even predicate the IPCC AR7 consideration of framing pathways. Here we suggest several policy research objectives that such a set of framing pathways should ideally fulfil, including mitigation needs for meeting the Paris Agreement objectives, the risks associated with carbon removal strategies, the consequences of delay in enacting that mitigation, guidance for adaptation needs, loss and damage, and for achieving mitigation in the wider context of societal development goals. Based on this context, we suggest that the next generation of climate scenarios for Earth system models should evolve towards representative emission pathways (REPs) and suggest key categories for such pathways. These framing pathways should address the most critical mitigation policy and adaptation plans that need to be implemented over the next 10 years. In our view, the most important categories are those relevant in the context of the Paris Agreement long-term goal, specifically an immediate action (low overshoot) 1.5 °C pathway and a delayed action (high overshoot) 1.5 °C pathway. Two other key categories are a pathway category approximately in line with current (as expressed by 2023) near- and long-term policy objectives, as well as a higher-emission category that is approximately in line with “current policies” (as expressed by 2023). We also argue for the scientific and policy relevance in exploring two “worlds that could have been”. One of these categories has high-emission trajectories well above what is implied by current policies and the other has very-low-emission trajectories which assume that global mitigation action in line with limiting warming to 1.5 °C without overshoot had begun in 2015. Finally, we note that the timely provision of new scientific information on pathways is critical to inform the development and implementation of climate policy. Under the Paris Agreement, for the second global stocktake, which will occur in 2028, and to inform subsequent development of nationally determined contributions (NDCs) up to 2040, scientific inputs are required by 2027. These needs should be carefully considered in the development timeline of community modelling activities, including those under CMIP7.
- Article
(2877 KB) - Full-text XML
- BibTeX
- EndNote
Having a core set of common pathways to drive Earth system models (ESMs) is essential for climate science, climate impact and climate policy communities. Such pathways are hereafter referred to as “framing pathways” since they provide a key set of consistent drivers (emissions, concentrations, land surface states, solar activity, etc.) for ESMs to build a range of climate futures which in turn provide a common framing input to conduct impact and vulnerability studies (Frieler et al., 2024; Warszawski et al., 2014). The framing pathways thereby provide a backbone of integration across the IPCC physical science (Working Group I) and impact (WG II) communities, as well as link to socioeconomic and mitigation information (WG III) (Fig. 1). Other avenues to integrate knowledge, such as global warming levels and cumulative emissions, referred to as additional “dimensions of integration” in IPCC AR6 (Fig. 1.24 in IPCC AR6 WG I) (IPCC, 2021), are also important, though the temporal and dynamic dimensions of scenarios are often vital both across biogeophysical and social domains to investigate climate system and impact responses. ESM simulations assessed in IPCC AR6 were largely conducted in the process of the Coupled Model Intercomparison Project Phase 6 (CMIP6; Eyring et al., 2016), with SSP framing scenarios prepared under a broad community effort and run by ESMs within the ScenarioMIP component of CMIP6 (Riahi et al., 2017; O'Neill et al., 2016; Tebaldi et al., 2021; Meinshausen et al., 2020; Gidden et al., 2019).
The choice of a core set of framing pathways yields influence well beyond the physical climate science and impact communities. Given its prominence in IPCC reports, as well as in the scientific literature (Riahi et al., 2017), scenario selection strongly influences the perception of what the climate science and policy communities understand as the range of plausible futures, feeding into climate risk assessments and informing the development of adaptation decisions and assessments of losses and damages, as well as the assessment of mitigation strategies and ambition. As an example, the inclusion of very-high-emission scenarios that are well above current policy projections in the core set of scenarios for CMIP6 has led to a continued focus on such a scenario in the literature and climate discourse that has come under criticism for mistaking a worst-case for a business-as-usual scenario (Hausfather and Peters, 2020; Huard et al., 2022; Box 3.3 in IPCC AR6 WG3, Riahi et al., 2022). For adaptation purposes, risk assessments and stress tests, those “high-end emission” pathways might continue to have some relevance as a proxy for “current policy emission, high-end climate response” pathways – in the absence of a systematic exploration of high-end climate sensitivity and carbon cycle feedback of “current policy” scenarios.
Scenario selection is not just of outstanding importance for climate science (e.g. favouring high signal-to-noise experiments). Climate science based on scenarios informs climate policy, and society in general, including uses by decision makers, the private sector and civil society, with examples ranging across broad areas such as climate litigation, financial risk analysis and regional adaptation planning (Rajamani et al., 2021; Richters et al., 2022; Otto et al., 2022). Such use cases need to be considered when designing a new framing scenario set. The assessment of scenario-based information is central to the IPCC in particular to provide climate information that is societally and policy relevant but not policy prescriptive. To support the IPCC in fulfilling this mandate, we argue that it is important that the scenarios run by ESMs cover a wide range of policy and physically relevant futures. That is mainly due to the unique position that ESMs play in IPCC assessment reports. They determine the boundaries of scenario exploration across various research communities, and hence the choice of pathways to run with ESMs is particularly crucial (because, by implication, any scenarios not covered by an ESM simulation will receive little, if any, attention). Not considering, for example, 1.5 °C-aligned scenarios would hamper a full information base for decision-making (Rogelj et al., 2018). On balance, both high-end and low-end emission scenarios are needed to explore carbon cycle and climate feedbacks, air pollution control, ecosystem consequences of overshoot (exceedance of and return below level of global warming) and “worlds avoided”.
1.1 Distinction between “framing climate pathways”, “socioeconomic pathways” and “scenarios”
The past use of different types of scenarios and pathways within the IPCC's assessment continues to create confusion even among well-informed stakeholders. The general understanding is that the IS92 scenarios (Leggett et al., 1992), SRES scenarios (Nakicenovic et al., 2000), representative concentration pathways (RCPs) and shared socioeconomic pathways (SSPs) are all scenarios through which plausible futures are investigated. While the term scenario is generally used as an overarching term, it is useful here to delineate between “scenarios” and “pathways”. Building on the definitional distinction in van Vuuren et al. (2014), we focus here on “pathways” that tend to be more unidimensional or narrow descriptions of a potential future, for example, a climate-related transient evolution of the future (emissions, concentrations and geophysical climate), without any explicit assumptions about socioeconomics or policy. In line with this definition, quantified socioeconomic futures, derived from qualitative socioeconomic narratives, can also be regarded as pathways on their own, as long as they are not integrated with “policy pathways”, “emission pathways”, “concentration pathways”, etc. Also following the definitional distinction in van Vuuren et al. (2014), we use “scenario” to refer to the combination of climate, socioeconomic and policy “pathways” into a coherent and internally consistent plausible future (Box 1). Note that the IPCC AR6 generally does not make such a distinction between the definitions of pathways and scenarios (see e.g. IPCC AR6 Glossary in van Diemen et al., 2022), reflecting the scientific literature more broadly.
We call the climate-focused descriptions of plausible futures “framing pathways” (see near-half white circle in Fig. 1). This naming highlights the relation to the RCP-type sets of emissions, concentrations and other biogeophysical drivers that are used to drive ESMs. Thus, this perspective on a new generation of pathways focuses on climate pathways only, and not on pathways for socioeconomic futures (e.g. population growth, GDP, etc.). Separating these two dimensions has precedent: the RCPs were developed in parallel to the SSPs (Moss et al., 2010; O'Neill et al., 2014). The motivation for this is that the climate modelling and climate impact communities can perform their simulations at the same time as the socioeconomic community determines narratives that are consistent with the climate pathways (RCPs in that case). This so-called parallel process (Moss et al., 2010) allows the different communities to work in parallel, reducing the time required to generate the outputs, increasing their cohesion as well as facilitating their assessment.
(On a side note, the generation of scenarios after the RCPs used again the SSP storylines and were called SSPx–y scenarios, where the “x” stands for the socioeconomic storyline and “y” for the RCP-like forcing level by the end of the 21st century. In other words, the socioeconomic storylines were kept “attached” to these key SSPx-y framework scenarios used in WG I, with both advantages and disadvantages.)
1.2 History and purpose of the “matrix” approach
The separation of socioeconomic and policy assumptions from climate change levels started with the split of the SRES A1 scenario family in 2001 (Nakicenovic et al., 2000). Back then, the high-technology-progress storyline of A1 was split into low-, medium- and high-emission scenarios. From the RCPs onwards, the so-called SSP–RCP matrix (Moss et al., 2010; van Vuuren et al., 2014) was used to explicitly present the climate and socioeconomic dimensions as independent dimensions. The different SSPs were represented by one dimension, while the climate outcome was represented by the other. Shared policy assumptions (SPAs) were employed to represent diverse policy assumptions, which led to varying emission levels for the same SSP (Kriegler et al., 2014). The SSPs themselves were constructed from two independent axes: challenges to mitigation and challenges to adaptation. The goal of the matrix approach was to allow different communities to assess a similar climate outcome while varying other dimensions (such as international co-operation, global economic growth, equity, adaptation, etc.). As some key aspects of the SSP–RCPs are becoming dated, it is now time to take stock of achievements and look for opportunities moving forward (O'Neill et al., 2020; Pirani et al., 2024).
While the SSP–RCPs were the foundation of IPCC AR6 WG I through ScenarioMIP (O'Neill et al., 2016), there was very little assessment of the SSP–RCPs in IPCC AR6 WG III (Riahi et al., 2022). From a mitigation perspective, the SSP–RCP framework used five SSPs across a range of forcing levels and was populated by six integrated assessment models (IAMs) (Riahi et al., 2017; Rogelj et al., 2018). These scenarios were important in IPCC SR15 (IPCC, 2018), but less so in IPCC AR6 WG III (IPCC, 2022a) as the scenarios started to become dated, did not explore policy-relevant alternative mitigation strategies, and were superseded by more recent literature. While the original SSP modelling exercise covered all the SSPs more or less evenly (Rogelj et al., 2018; Riahi et al., 2017), the SSP2 “middle of the road” scenario has since been used by most modelling groups as a default socioeconomic pathway and represents more than 90 % of the 1202 scenarios with a climate assessment in the IPCC AR6 WG III scenarios database (Riahi et al., 2022). The SSP–RCP framework also focused on particular challenges (mitigation and adaptation) and had limited scope to address other contemporary questions such as temperature overshoot (Riahi et al., 2022), equity (ENB, 2023; Kanitkar et al., 2024) or de-growth.
While the climate-focused framing pathways, the focus of this paper, should largely be treated as separate from these socioeconomic dimensions, it is in our view essential that we assess and explore socioeconomic considerations over the coming years, and any scenario framework needs to consider ways to ensure that these aspects can be assessed. In other words, having a common set of geophysical framing pathways needs to be followed by an exploration of multiple socioeconomic dimensions (adaptation, impact, equity, finance, etc.) and potentially also by normative choices. For cases where the influences of different socioeconomic futures are investigated, common practice seems to be that impacts, adaptation and vulnerability communities use a single climate outcome (such as the one from SSP2-4.5) while ex-post varying other assumptions such as population, income and inequality (rather than exploring SSP1-4.5 or SSP3-4.5, which were not evaluated by ESMs in the last phase of ScenarioMIP). The exploration of different socioeconomic dimensions is, however, not the bottleneck in physical climate science in the immediate future, as they are not an input to the ESMs. The bottleneck is the computationally expensive ESMs and hence the need to focus on, and prioritise, a set of a few geophysical framing pathways. Those framing scenarios can be implemented in a coordinated manner by international ESM modelling centres, and subsequently, the output of these ESMs can be married with a variety of socioeconomic futures to assess vulnerability, impacts and adaptation challenges. We see this proposal not as a contradiction but rather an evolution of the SSP–RCP approach to account for a greater variety of socioeconomic futures. Yet, in order to generate the forcings for our framing pathways, initial assumptions about socioeconomic development and consistent modelling in integrated assessment models will be required, in particular for localised forcings such as aerosols or land use change. This may constrain the extent to which socioeconomic dimensions can be varied while remaining consistent with the forcing pathways provided.
1.3 High level framing and history
During past IPCC assessment cycles, scenario expert meetings in 2007 in Noordwijkerhout (IPCC, 2008) at the end of the AR4, and 2015 in Laxenburg (IPCC, 2016) at the end of AR5, provided recommendations that informed the selection of the key scenarios that were run as part of the fifth and sixth phases of CMIP5 and CMIP6 with ESMs. Specifically, the 2007 Noordwijkerhout IPCC expert meeting decided to extend the lower bound of scenarios towards mitigation scenarios (which was a departure from the 2001 SRES set of scenarios that only covered non-climate-policy scenarios) by including the so-called RCP3-PD pathway, which can be loosely regarded as a scenario that leads to approximately a “below 2 °C” warming and for the first time considered net negative CO2 emissions in the second half of the 21st century. In March 2015, the IPCC Laxenburg expert meeting again pushed the envelope in order to be policy relevant, given that much of the policy and impact discussion had shifted to lower warming levels, specifically 1.5 °C. Thus, a so-called SSP scenario with a radiative forcing outcome of approximately 1.9 W m−2 by the end of the century was added to the set of key scenarios. This allowed CMIP6 to include climate model and ESM runs that were intended to approximately align with the Paris Agreement long-term temperature goal – agreed by the end of 2015 – of pursuing efforts to limit warming to 1.5 °C. The IPCC concluded its Sixth Assessment cycle (AR6) with a workshop in Bangkok in April 2023 (IPCC, 2023) that covered lessons learned in the AR6 and recommendations for the AR7 and future IPCC assessments of scenarios. Part of the agenda was a breakout group on “recommendations for the scientific communities involved in modelling”, although – unlike at other IPCC scenario workshops – no recommendations were made on the specific point of the future scenario design in terms of forcing or warming levels. The workshop report reflects the discussions held at the meeting, i.e. “It will be useful to explore differences between high overshoot (C2) and limited overshoot (C1) across WGs, enhancing policy relevance”. The need to inform the second global stocktake under the Paris Agreement in 2027–2028 was also highlighted (IPCC, 2023). The workshop called on the larger scientific community to provide input, which is the motivation for this paper.
1.4 Towards representative emission pathways
In this perspective we identify categories that could inform the development of framing climate pathways for ESMs based on expert and stakeholder discussions across various communities. Those discussions started during the AR6 cycle and continue with the open participation and review approach used to develop this paper and will be held in multiple other fora as well. As a tentative name, we suggest “representative emission pathways” (REPs). The basis is a close alignment with the RCPs of CMIP5 and the RCP component of the SSP–RCP matrix. Because future ESM simulations may be predominantly emission driven (for at least carbon emissions) to capture carbon cycle uncertainties, we suggest changing the term from “concentration” to “emissions” so that the new generation is called “representative emission pathways” rather than “representative concentration pathways”. The shift to an emissions-driven framing encourages exploration of additional degrees of freedom in scenario definition: regional aerosol emissions, land use strategy and carbon removal where process representation in ESMs can add to understanding (Sanderson et al., 2023), but it does not restrict the ability to perform concentration-driven simulations.
To enable the strong participation of the full set of ESMs, including those that are not yet capable of being emission driven for CO2, CH4 and N2O, and to incorporate the effect of many other climate forcers for which reduced complexity models remain a more efficient choice in terms of computing time, “best-estimate” concentration forcings would be available to accompany the REPs. The projection of concentrations (either a single trajectory or a range) could be a reflection of the best-estimate expert judgement in the most recent IPCC assessment or a more recent expert judgement (such as including new insights, e.g. natural CH4 emission dynamics in wetlands; Kleinen et al., 2021) and could be produced by calibrated emulators (Cross-Chapter Box 7.1 in IPCC AR6 WG I; Forster et al., 2021). The input data provision for ESMs could even be extended by providing high and low concentration projections for the REPs, so that even ESMs that cannot start from CO2, CH4 or N2O emissions could include the gas cycle uncertainty to some extent, if desired.
While REPs will most likely be derived from integrated assessment models (IAMs), we argue that the REPs should remain separated from the underlying socioeconomic scenarios as was done previously under the RCPs (Moss et al., 2010). We recommend that such a separation be essential to enhance uptake and facilitate exploration of alternative socioeconomic and other dimensions by adaptation, equity, finance and other scientific communities outside the geophysical science domain.
While ideally non-CO2 forcers will also be included at the point of emissions and precursors, future ESM simulations will continue to implement some of those in terms of concentration or abundance inputs (N2O, hydrofluorocarbons (HFCs), perfluorocarbons (PFCs), ozone-depleting substances (ODSs) and aerosols) in acknowledgement both of ESM capacities and computational efficiency. The spatially heterogeneous emission and abundance fields of short-lived forcers will depend on the socioeconomic background assumptions, and while our perspective emphasises a separation of the socioeconomic narratives and emission pathways like the one pursued under the RCPs, the specific short-lived forcer emission and abundance fields will be dependent on those background socioeconomic choices. A specialised MIP could investigate how climate outcome depends on socioeconomic pathways that are underlying integrated assessment models with the same (or similar) global emission pathway outcome. Anyway, for those heterogeneously emitted and short-lived species, we acknowledge that one limitation of the SSP–RCP framework continues to hold in the sense that a full and clean separation between REPs and socioeconomic pathways (that were assumed for the generation of these REPs) is not possible to the same extent as for well-mixed greenhouse gases.
1.5 REPs are only a small part of the overall scenario spectrum
We reiterate that these framing climate pathways are only a small subset of the scenarios that play an important role in IPCC assessments. Working Group III investigates thousands of socioeconomic pathways from the independently generated scientific literature, including their sectoral, national, regional and socioeconomic dimensions. Even in AR6, the SSPx–y scenarios did not feature prominently in WG III, where it was rather the so-called illustrative mitigation pathways (IMPs) that highlighted choices about technology, infrastructure and behavioural responses. As frequently pointed out in the IPCC approval sessions, it is also important to consider the equity dimension of scenarios. These points imply a renewed effort to cross-examine each of the future climate change levels under a broad range of socioeconomic futures. At this stage, while developing REPs as input forcings for ESMs, it is not necessary to finalise or pre-empt the scope of socioeconomic futures and national level scenarios, given the importance of exploring a greater diversity thereof. A task of future IPCC reports will be to reflect the whole spectrum of research on socioeconomic futures and national scenarios. The need to coalesce on a set of framing climate pathways for the ESMs only arises due to the ESMs' multi-year lead times and high computational costs (Moss et al., 2010). But even for ESMs, a set of framing scenarios does not pre-empt a number of additional investigations, e.g. to allow for both, individual modelling groups to pursue their own scenarios or new intercomparison projects to be added later, such as ZECMIP in the CMIP6 cycle (Jones et al., 2019; MacDougall et al., 2020). We do not expect the REPs to be the only reflection of scenario issues in the wake of the just-concluded IPCC AR6 – with the ultimate aspiration being that the next cycle, AR7, will have a broad range of suitable ESM scenarios from which to select – from a policy-relevance, climate services and scientific point of view (see e.g. Pirani et al., 2024).
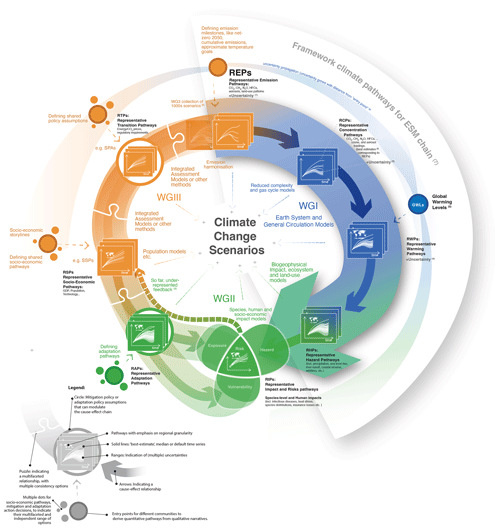
Figure 1Our conceptual overview of climate change scenarios, representative emission pathways (REPs) and framework climate pathways. Scenarios are constructed from socioeconomic, emission, concentration and impact pathways that are internally consistent with each other (“+” in middle). We propose anchoring the input for ESMs around “Representative Emission Pathways” (REPs) (“1”), in contrast to anchoring around RCPs as was done in IPCC AR5 and IPCC AR6. However, the intention is to flexibly allow different starting points for different communities. For the gas cycles that are not represented in some ESMs, reduced complexity models can translate emission pathways to concentration pathways. The “best-estimate” (“2”) concentration projections in line with REPs can be labelled RCPs, although the “representative pathways” across different subdomains (emissions, concentrations and socioeconomic) do not necessarily have to be consistent. Furthermore, different models and approaches would translate emissions to concentrations differently, spanning an uncertainty range (“3”). “Representative Warming Pathways” (RWPs) (“4”) can provide another entry point into scenario design, closely aligned by a consideration of “Global Warming Levels” (GWLs) (“5”). If derived from REPs, the range of derived warming pathways would represent both gas-cycle and climate feedback uncertainties (“4”). Biogeophysical hazard models, ecosystem and land use could then be driven by the output from ESMs to produce hazard pathways. Potentially, one could also design representative hazard pathways (RHPs) either as best-estimate representations of REP's hazards or independently. The segment of the cause–effect chain from emissions to hazards, computed by a chain of climate and other numerical biogeophysical models, is called here the “Framework climate pathways for ESM chain” (bold arrow on right side on white circle segment and “7”). Taking into account adaptation options, potentially as “Representative Adaptation pathways” (RAPs), the so-called RIPs (“Representative Impact and Risks pathways”) could be derived, taking into account vulnerability and exposure, with a full uncertainty propagation from REPs now spanning a wide range for each starting REP. Another entry point into scenario design are socioeconomic narratives, which can be translated into quantitative socioeconomic pathways. In this terminology, the quantitative shared socioeconomic pathways (SSPs) (O'Neill et al., 2014; Riahi et al., 2017) would be termed “representative socioeconomic pathways” (RSPs). Those socioeconomic pathways would ideally take into account some climate impacts (dashed curve, “8”), an under-represented feedback so far. In combination with mitigation policy assumptions (such as the shared policy assumptions; Kriegler et al., 2014), which are here coined “Representative Transition Pathways”, integrated assessment models can derive a large set of emission pathways (“9”) to investigate mitigation options. Even within this diagram and current processes, there are still factors, such as socioeconomic inertia and psychosocial delays in implementation of climate mitigation and adaptation actions, that are not currently considered.
In the subsequent sections, we first lay out several design criteria and needs from a policy point of view that, in our opinion, geophysical framing scenarios should ideally meet. Subsequently, we provide an overview, from a scientific point of view, of design criteria that geophysical framing scenarios should meet. We then identify pathway categories that could inform the design of REPs to meet these policy and scientific objectives within the constraints of a potential scenario ensemble CMIP7 design.
The decisions of the United Nations Framework Convention on Climate Change (UNFCCC) and the Paris Agreement, as well as subsequent decisions, are central to the policy context. The new set of framing climate pathways would need to meet the related key mitigation (Sect. 2.1), along with adaptation as well as loss and damage information needs (Sect. 2.2), by addressing, to the extent possible, important policy-relevant questions collated below in a non-comprehensive list (Sect. 2.3).
2.1 Focus on Paris Agreement relevant scenarios from mitigation decision-maker viewpoint
The elements outlined in the Paris Agreement, more specifically in relation to the long-term temperature goal expressed in Article 2.1 and Article 4.1, should be fully explored (Schleussner et al., 2022). Under the UNFCCC, the second periodic review of the long-term global goal under the Convention, and of overall progress towards achieving it, concluded its work in 2022. Furthermore, the global stocktake provides a checkpoint on whether aggregate emission levels by parties to the agreement are consistent with the long-term goals of the Convention (UNFCCC, 2022a). The Conference of the Parties under the UNFCCC explicitly “acknowledges that limiting the global average temperature increase to 1.5 °C above pre-industrial levels with no or limited overshoot would avoid increasingly severe climate change impacts” (UNFCCC, 2022b), building on the conclusions of the IPCC “Special Report on Global Warming of 1.5 °C” (Hoegh-Guldberg et al., 2018), as well as the IPCC AR6 WG I and WG II reports (IPCC, 2022b, 2021). They also concluded that while “information and knowledge have improved significantly since the first periodic review (2013–2015)” (UNFCCC, 2022b, paragraph 7), “there continue to be important information and knowledge gaps” in relation to its scope, including on “the long-term global goal and scenarios towards achieving it in the light of the ultimate objective of the Convention”. The scientific community is explicitly encouraged to address those gaps. We are of the view that any scenario design process needs to be cognisant of this explicitly expressed call by governments for more scientific information on Paris Agreement compatible scenarios.
Against a backdrop of “the emission world avoided” context provided by high-end emission scenarios, it is paramount that decision makers also understand the implications of stronger mitigation efforts in terms of climate benefits and avoided impacts. Whether we follow a scenario that delays mitigation efforts by 10, 20 or 30 years and reaches net-zero CO2 emissions by 2050 or 2060 or 2070 makes trillion-dollar differences in terms of directing government incentives and private capital (Riahi et al., 2022; van der Wijst et al., 2023), but also in terms of adaptation costs, limits to adaptation, irreversible loss, as well as economic and non-economic costs of anticipated losses and damages (IPCC, 2022b). While natural variability in any single year influences global-mean temperatures by ±0.25 °C (Box 4.1 in IPCC AR6 WGI; i.e. Lee et al., 2021), climate extremes (Seneviratne et al., 2021) and impacts that reflect long-term, cumulative climate changes (e.g. glacier melt or sea level rise) can be substantially different between a scenario peaking at 1.6 or 1.8 °C in the middle of the century (Mengel et al., 2018; Pfleiderer et al., 2018). Only immediate action will slow anthropogenic warming in the near term (McKenna et al., 2021), a crucial element to enable sustainable development (Schleussner et al., 2021).
2.2 Comprehensive range to inform risk management, adaptation needs, as well as loss and damage assessments
Output from ESMs based on the framing pathways should explore a comprehensive range of plausible warming futures. Plausible very-high-end or worst-case outcomes are a key foundation for risk management and adaptation decision-making. Also, those high-end outcomes can be indicative of the high-end tail of the distributions, including high-end climate sensitivity, that are helpful in examining limits to adaptation that may need to be addressed. Information about high-end global warming outcomes is of particular importance on adaptation- and loss-and-damage-relevant timescales, i.e. up to 2050. It is important to be cognisant of the fact that on adaptation-relevant timescales until mid-century, climate uncertainties dominate scenario uncertainty (Lehner et al., 2020; Lee et al., 2021). As such, even a very-high-emission pathway would not allow for a full appraisal of high-end outcomes on those timescales. Rather, an assessment of high-risk outcomes needs to be based on assessing higher risk percentiles of any given pathway.
In principle, there are two options for examining the high-end warming futures for these purposes: use a high-end pathway, such as “the emission world avoided” scenario proposed here (e.g. SSP5-8.5 or SSP3-7.0), and then – by examining impacts at different global warming levels (GWLs) – map climate characteristics onto the high percentiles of expected tails of the distribution of lower-emission pathways, such as the “current policy” pathways. Alternatively, use the higher-end warming tails of the ESMs that were run for those “current policy” pathways, acknowledging that a lack of high ensemble sizes and a lack of a complete representation of uncertainties might hinder a full examination of the tails of the projected distribution.
In contrast to the high end of the scenario space, the plausible and very low end of the scenario space is a prerequisite to deriving minimal adaptation needs and identifying unavoidable loss and damage. Understanding the very low end of the scenario space is also key to understanding the consequences of delayed action, i.e. what we are leaving behind or taking off the table. Thus, a useful framing pathway is one in which immediate action had started in 2015 and that has at least a 50 % probability of decadal average temperatures remaining below 1.5 °C.
2.3 Non-exhaustive list of policy-relevant questions
We postulate a non-exhaustive list of policy-relevant questions. The degree to which the framing pathway design can address those questions or not will influence to what degree the framing climate pathway dataset can inform the forthcoming seventh assessment cycle of IPCC (AR7) and the wider scientific literature based on CMIP7 to be policy relevant.
- a.
What are the potential climate outcomes of current climate policy targets? The climate ambition reflected in the current set of nationally determined contributions (NDCs) is insufficient to meet the Paris Agreement temperature goal (Riahi et al., 2022; Meinshausen et al., 2022). However, beyond the near term, different interpretations of the stringency and credibility of expressed net-zero targets provide for a broad range of different emission trajectories and subsequent warming outcomes ranging from projected warming between 2.5 and 3 °C by 2100 (and continuation thereafter) to a pathway in which median peak warming is limited to less than 2 °C (Rogelj et al., 2023). Exploring this range is key to inform the implementation and refinement of established targets.
- b.
What mitigation is required to limit warming to around 1.5 °C and what climate impacts can still be avoided? The design criteria would be strong mitigation consistent with the Paris Agreement and national targets (such as net-zero CO2 by 2050). Only by exploring such a lower-emission future in the ESMs can science support integrated and informed decision-making to pursue synergies of mitigation and adaptation actions consistent with the Paris Agreement. It might be that such a pathway with net-zero CO2 emissions around the middle of the century, limited cumulative emissions until then and net-zero GHG emissions in the second half of the century (as Article 4.1 of the Paris Agreement aims to achieve) is at the very low end of future emissions that some integrated assessment models can produce. ESMs themselves would also help inform confidence in the lower warming bound, providing a process-driven representation of the climate outcome of a maximally strong decarbonisation and land use strategy proposed in the scenario. Such low-end scenarios are paramount to estimate what the remaining carbon budget is and what climate impacts might still be avoidable, as well as to inform questions of climate justice. (The low-end emission pathways are assumed here to be loosely defined by the maximal speed of the transition towards a net-zero future (and beyond) from a technological and resourcing point of view – which will differ in each IAM model's implementation of the latest cost, technology and deployment information. This lower bound is subject to uncertainties and definitional choices.)
- c.
Within the range implied by the Paris Agreement temperature goal, what are the different feasible mitigation strategies? The extent to which a temporary overshoot above global warming of 1.5 °C, always constrained by holding warming to “well below” 2 °C, is regarded to be in compliance with the Paris Agreement differs among different policy stakeholders (e.g. Mace, 2016). While some interpretations have been suggested (Schleussner et al., 2022) the exact definition of “well below” 2 °C has also not been established in the policy domain. Not pre-empting those decisions requires a set of scenarios with different median peak warming broadly within the range of temperature levels referred to in Article 2.1 of the Paris Agreement (i.e. between 1.5 °C or below and less than 2 °C). This might include distinguishing peak-and-decline pathways from stabilisation pathways at 1.5 °C, or at levels above 1.5 °C but less than 2 °C.
- d.
What are the interlinkages of Paris Agreement compatible climate action and a broader sustainability agenda? Climate and environmental policy aim for a broader set of sustainability objectives beyond emission outcomes alone. Many of those interlinkages with sustainable development goals (SDGs) are explored in the framework of different SSPs, but insofar as they relate to different land use futures will also be of direct relevance for climate outcomes. Global sustainable land futures will be decisively different from a continuation of unequal trends, both in terms of GHG emission trajectories and also for land-cover change, water and fertiliser use, biodiversity, etc. (Humpenöder et al., 2022). Exploring such sustainable scenarios is directly relevant not just for informing climate policy but also in other fora such as the discourse on biodiversity.
- e.
What are the consequences of delaying mitigation? In the Glasgow Climate Pact, countries recognised the need for accelerated action in this critical decade (UNFCCC, 2021). However, a yawning gap remains between 2030 emission levels implied by current nationally determined contributions under the Paris Agreement (as of 2023) and 1.5 °C pathways (IPCC, 2022a). To inform the ambition expressed in the Glasgow Climate Pact, robust scientific information on the consequences of delaying stringent climate action is highly policy relevant. Without both an immediate and a delayed scenario that aim to reach, for example, 1.5 °C by the end of the century, policymakers will not be able to obtain relevant information on the consequences of delay from dedicated impact studies that are dependent on ESM simulations. These ESM simulations will be fundamentally important to enable, for example, the impacts and adaptation limits assessment of IPCC AR7.
- f.
Non-CO2: what are the effects of non-CO2 mitigation? Addressing non-CO2 GHGs, in particular methane, has risen in prominence in policy circles. For example, the Global Methane Pledge, supported by more than 150 countries, specifically focused on stringent methane emission reductions by 2030. Distinguishing between different emission strategies with focus on shorter- or longer-lived GHGs, and/or air pollution (namely aerosols, ozone and their precursors) reduction, is policy relevant. Within CMIP5, RCP-driven simulations, non-CO2 emissions and, in particular, the SO2 emissions were rather closely aligned across the low- to high-end scenarios. The SSPs somewhat corrected for this with consideration of various air pollution controls derived from the overarching SSP narrative and, as a result, show a wider variation across both non-CO2 GHGs (and their precursors) and aerosols (see e.g. Cross-Chapter Box 1.4 and Fig. 2 in IPCC, 2021; see also Gidden et al., 2019). However, the sustainable pathways included both strong climate change mitigation and air pollution control preventing disentanglement of the co-benefits of climate change mitigation policies from actions focused on air pollution (Szopa et al., 2021). It would be useful to investigate the implications of different gas-to-gas emission strategies, including regionally varying mitigation policies to capture geographical patterns of short-lived climate forcer emissions, both in the framework scenarios as well as in dedicated sensitivity scenarios in additional MIPs (Persad et al., 2022, 2023). Generally, examining the flexibility governments have to combine mitigation strategies for CO2 and non-CO2 GHGs, as well as air pollutants, in terms of climate outcome consequences, is of interest – aiming for a more encompassing reflection of non-CO2 atmospheric interactions and uncertainties. Assessing the co-benefits of climate-driven policies on air pollution can be important for decision-making. These latter aims also rely on the emerging capability of some ESMs for non-CO2 greenhouse gases to be emissions driven (Folberth et al., 2022).
- g.
Land-based carbon dioxide removal: what are the relative risks and the effectiveness of different negative-emission strategies? RCP-based designs do not allow significant uncertainties around land-based carbon dioxide removal to be assessed, because emission fluxes are calculated in the context of IAMs using semi-empirical land carbon accounting that does not represent climate impacts on the strength of the land and ocean carbon sink, and on nature-based solutions overall (IPCC, 2022b). Complex carbon process representations used in land surface components of ESMs are increasingly able to capture dynamics associated with carbon fertilisation effects, plant demographic responses, heat stress, drought response and fire risk, all of which could potentially radically alter the capacity for land-based negative-emission fluxes. Defining scenarios in terms of fossil emissions and land use patterns or decisions allows these processes to be represented directly.
- h.
Overshoot: to what extent are climate change and the impacts of climate change reversible, and on what timescales? Significant differences in mitigation requirements in the second half of the century between different classes of overshoot scenarios have been identified in the latest IPCC assessment (for example, as reflected in IPCC WG3's C1 and C2 categories (Riahi et al., 2022)). Information on the climate system consequences and related impacts of overshoot, however, is not comprehensively available (Asaadi et al., 2024; Santana-Falcón et al., 2023). Exploring the differences between different policy-relevant overshoot scenarios with ESMs is critical to address this gap. This would also enable policy-relevant information regarding the ways in which envisaging declining future temperatures might influence policy choices today, while temperatures are still rising. Any co-benefits and more ancillary impacts of future negative-emission options (beyond land-based carbon dioxide removal) require an enhanced focus on multiple options to limit warming to 1.5 °C in the long term.
- i.
Providing a backdrop of “the world that could have been”. To calculate benefits of past and future climate action and inaction, it is useful to have hypothetical backdrop scenarios, both at the high and the low end. The “world that could have been” at the high end could be a medium/high-emission scenario, such as SSP3-7.0 from the CMIP6 cycle – depicting a future that unfolds in the absence of climate policies and in the absence of economic shifts that were largely initiated by climate policies (like “learning-by-doing” renewable technology cost reductions). At the low end, a “world that could have been” can provide a hypothetical backdrop of what would have been still possible if countries had acted fast enough to avoid 1.5 °C warming at all times from 2015 onwards (when the 1.5 °C goal was first adopted within the UNFCCC context in the Paris Agreement). Such scenarios will be vital to inform an emerging policy discussion around loss and damage under the Paris Agreement, as it provides a reference point of the loss and damage that could have still been avoided.
- j.
What could a worst-case-outcome world look like? Due to different types of uncertainties (biogeochemical but also societal and geopolitical), it is important also to understand a low-likelihood but high warming outcome (Lee et al., 2021). A more elaborate way to explore the tails of the warming distributions of the scenarios seems warranted (Kemp et al., 2022).
- k.
What are the climate effects of different regional emissions? For shorter-lived air pollutants, in particular aerosols, as well as for land use changes, regional climate effects can markedly differ for geographically variable forcings, and emissions from various regions will have different regional and global implications (Seneviratne et al., 2018b; Persad et al., 2022). In addition, some short-lived forcers have physically distinct mechanisms of interaction with the global climate, such as directly absorbing sunlight or altering clouds, perturbing precipitation and large-scale circulation, and have effects distinct from those of well-mixed GHGs (Tang et al., 2018; Liu et al., 2018; Sillmann et al., 2019; Persad et al., 2023).
- l.
What is the time dependence of climate change impacts? How does the climate evolve even under near-stable global temperatures? Net-zero CO2 emissions are expected to result in slowly changing global temperatures (King et al., 2021; MacDougall et al., 2020), but understanding of regional climate and long-term biogeophysical impacts of net-zero CO2 emissions remains limited. In the literature, there is currently a large dependence of global-warming-level-based projections on sampling from fast-warming scenarios such as SSP5-8.5 (e.g. AR6 Interactive Atlas). Exploring how regional climates and different aspects of global climate evolve at a stable Paris Agreement-aligned global warming level (e.g. Sigmond et al., 2020; Mengel et al., 2018; Seneviratne et al., 2018a) would improve understanding of the effects of climate stabilisation and inform policy- and decision-making.
- m.
What are the long-term implications beyond 2100? Scenarios supplied by IAMs have focused on a time horizon of 2100, which has been consistent throughout the evolution of scenarios from SRES to RCPs to SSPs. In the early 2000s this was considered appropriate, but 2100 is now less than one human lifespan into the future and within the life cycle of a lot of new infrastructure (Lyon et al., 2022). Several Earth system consequences, including sea level rise (Mengel et al., 2018), ice sheet loss and carbon cycle dynamics (Koven et al., 2022), as well as impacts on the natural system (Santana-Falcón et al., 2023), will continue beyond 2100, even in strong mitigation scenarios. While RCPs and SSPs included extended scenarios for running ESMs beyond 2100 (Meinshausen et al., 2011, 2020), they were not based on detailed scenario modelling provided by the IAM community and, particularly in CMIP6, had low take-up among modelling groups (Lee et al., 2021). Irreversibility is also coupled with the question of overshoot within this century and beyond (Frölicher and Joos, 2010).
In this section we focus on key scientific questions to guide design of future framing pathways. A much broader reflection on a vision for the future of climate modelling is, for example, expressed in a recent WCRP 2022 workshop and its meeting report (WCRP, 2023). This vision explicitly identifies a priority for co-design approaches with users and key partners, including the IPCC and other assessment communities, and focuses on deep mitigation scenarios for ESM scenarios.
Several key scientific frontiers can be identified that, in some cases, overlap with the policy questions identified above. From our perspective these frontiers include, but are not limited to, those listed below. Many of the scientific advances can be expected from specific sensitivity pathways that are more hypothetical in nature, such as excluding or including a certain forcing agent, pulse response, abrupt change and other idealised experiments. Thus, many of the scientific advances can be expected to emanate from the specialised MIPs (CFMIP, HighResMIP, AerChemMIP, C4MIP, RFMIP, CDRMIP, GeoMIP, LUMIP, ISMIP, OMIP, VolMIP, DAMIP, etc.; see https://wcrp-cmip.org/mips/, last access: 3 May 2024) that are conducted in parallel to running ESMs with the multi-gas scenarios.
Some scientific questions are, however, also particularly relevant for the design of framing pathways. Those scientific research questions include the following:
- a.
What is the timescale of emergence of mitigation benefits? While questions of overshoot and zero emission commitment (ZEC) relate to long-term climate outcomes, the question of the emergence of mitigation benefits in the near term is important to adaptation as well as loss and damage policy. Outlining and understanding when, how and which benefits of mitigation emerge is the basis to inform what impacts of climate change can still be avoided (Ciavarella et al., 2017; McKenna et al., 2021; Samset et al., 2020). This requires specific focus also on mitigation of non-CO2 GHGs (Lanson et al., 2022; Samset et al., 2020), the regional climate effects of aerosols (Persad et al., 2022), disentangling the effects of air pollution policies from GHG emission reduction policies and a more clearly defined non-mitigation counterfactual scenario. Ideally, many individual sensitivity scenarios for individual forcers would be undertaken to investigate the emergence of climate effects due to mitigation action on individual forcers, but the overall framework design can assist in quantifying an aggregate effect of multi-gas mitigation action.
- b.
What is the zero emission commitment (ZEC)? One of the most central science-based benchmarks for climate policy is the focus on achieving net-zero CO2 emission targets to halt global warming. While no further warming for net-zero CO2 emissions is a robust central estimate identified in the AR6, the uncertainties around that central estimate remain substantial, as well as how the zero emission commitment might change as a function of cumulative emissions at the point of net-zero CO2. A very robust understanding of this zero emission commitment (Jones et al., 2019; MacDougall et al., 2020; Palazzo Corner et al., 2023) and how it relates to realistic net-zero transitions should therefore be a central objective of CMIP7. Likewise, a robust understanding of the implications of achieving and sustaining net-zero GHG emissions (as part of Article 4.1 of the Paris Agreement) under the global warming potential (GWP)-100 metric would be a useful and very policy-relevant insight (Schleussner et al., 2022).
- c.
Climate system and carbon cycle feedbacks, also under overshoot scenarios. Whether or not global temperature increase is indeed reversible strongly depends on the response of the climate system and the carbon cycle to a decisive reduction in radiative forcing (Schwinger and Tjiputra, 2018; Melnikova et al., 2021). Some feedbacks, such as permafrost melt, will continue over centuries even if warming is reversed following overshoot (Gasser et al., 2018; Asaadi et al., 2024). More generally, the response of natural emissions is highly uncertain and can also drive the evolution of several non-CO2 climate forcers with consequences for biogeochemical climate feedbacks and health, threatening to counterbalance the air pollution control efforts in some places (Szopa et al., 2021). At the same time, the impacts of carbon dioxide removal (CDR) methods on the carbon cycle need to be examined by ESMs running these overshoot scenarios (Melnikova et al., 2021), as well as any “reversal risks” to carbon stocks that were enhanced under CDR actions. A systematic exploration of those feedbacks is required to critically assess the potential and risks under overshoot scenarios.
- d.
Which changes in the climate system are reversible and which are not (hysteresis)? An emerging body of science indicates that impacts of climate change will continue beyond halting global warming or even overshoot. This is most likely for time-lagged systems such as sea level rise (Mengel et al., 2018), but also potentially for circulation patterns, rainfall and climate extremes (Pfleiderer et al., 2023). The question of whether and under what conditions irreversible thresholds of ice sheets or other systems may be crossed is also key (Wunderling et al., 2023). This is increasingly not a question of comparing very high to low warming scenarios, but emerging evidence suggests significant increases in risks between 1.5 and 2 °C that require this range to be resolved very thoroughly.
- e.
How do biophysical consequences of land-based CDR compare with potential impacts avoided under overshoot scenarios? The impact of wide-scale land-based CDR (e.g. afforestation, biomass production and enhanced weathering) for land surfaces will reach beyond impacts on biodiversity and food security, and can also contribute to changing albedo and non-CO2 emissions (Fuss et al., 2018), or regional weather patterns (Pfleiderer et al., 2023). Global warming and related impacts on terrestrial ecosystems and their uses, particularly on their disturbances such as fires, droughts and pests (Westerling et al., 2006; Liu et al., 2023; Canadell et al., 2021), can also influence the durability of these CDR interventions in ways that are poorly represented in current IAMs. Investigating futures with ESMs in which more or less emphasis is placed on offsetting residual emissions with CDR can provide insights into the implications of some mitigation or removal strategies.
- f.
Fidelity of ESMs against observed climate change. Understanding emergent constraints had a central role in IPCC AR6 in constraining future projections (Brunner et al., 2020; Tokarska et al., 2020; Ribes et al., 2021; Liang et al., 2020). The historical realism of CMIP7 ESM simulations will be key to scanning different forcing agents and their (combined) effects, and will likely require an iterative process. To what degree are performance metrics, e.g. the agreement of ESM output with observations (Hajima et al., 2024), useful in learning about global and regional futures?
- g.
Change in ESMs from generation to generation. A clear comparison point to measure advances and differences in ESMs from CMIP5 to CMIP6 was missing, as the underlying scenarios differed substantially. This proposal could address the need for having at least one overlapping scenario by using a previous generation scenario as the “the emission world avoided” (TEWA) scenario, such as SSP3-7.0 or SSP5-8.5, for example. This strategy would also allow for extended time for climate impact assessment, which has been historically difficult to achieve in the timeline between the delivery of ESM scenarios and the IPCC assessments (WG II in particular, as noted in Pirani et al. (2024).
The impression could arise that the policy and scientific objectives relevant to the framing pathways are in conflict. While a policy-relevant question almost always entails a scientific question of interest, the scientific realm of questions is broader. For example, the policy interest in the differences, in terms of impacts, between pathways with low and medium overshoot of 1.5 °C scenarios also includes interesting scientific challenges, e.g. how to quantify, in more detail, the IPCC finding that “every bit of warming matters”, using, for example, new statistical techniques to detect climate change signals (e.g. Sippel et al., 2020). Staying with this example, previous designs of the framing pathways did not provide the opportunity to investigate the extent to which we can detect signals that might, at first sight, be considered too small given the size of natural variability.
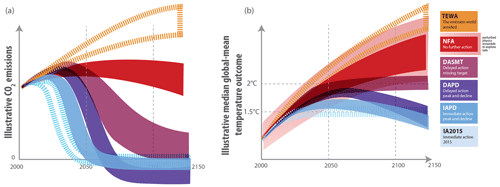
Figure 2Sketch overview of framing pathway categories for ESM simulations. Illustrative CO2 emission trajectory ranges and corresponding global-mean temperature outcomes are shown in a and b, respectively. The temperature outcomes are approximate median outcomes under IPCC AR6 assessed climate characteristics. In predominantly emission-driven runs, the range of temperature outcomes would vary even further across models, because pathways are defined by their carbon emissions in conjunction with forcing series for non-CO2-driving forcers and because different models exhibit different responses. Categories that represent hypothetical edge pathways (“the worlds that could have been”) are possibly of lower priority (illustrated by dashed lines) but frame the pathway space at both the higher-emission end and lower-emission end. The NFA category pathway should ideally also be run by perturbed physics ensembles and other approaches to capture the full uncertainty and its tails of warming so that a higher-warming-outcome representation of that REP could serve as a proxy for high-impact, low-likelihood scenarios (“see background red range” for NFA category). This would be particularly important for adaptation and risk assessment. If a perturbed physics ensemble high-end warming outcome of the NFA scenario is not able to be investigated, the high “the emission world avoided” TEWA category could also provide a proxy for a high-end/worst-case warming outcome under lower-emission futures.
Framing pathways for ESMs under the CMIP initiative should ideally address the policy-relevant and research-oriented questions discussed above. In the following, we describe key characteristics and categories of framing pathways that could inform their selection. Key characteristics are as follows:
- a.
Explore the full range of long-term outcomes of these pathways under overshoot and time-lagged feedbacks this century and beyond. A default extension of scenarios to 2150 may be pragmatic while providing meaningful, and potentially diverging, extensions on even longer timescales (until 2500) to explore a range of different very-long-term futures, including long-term temperature decline scenarios.
- b.
Pathways should be emissions driven and land use strategy driven for CO2. Concentration-driven pathways can still be included in the wider CMIP effort to allow for a more systematic intercomparison of emission- and concentration-driven approaches. Non-CO2 GHGs, especially CH4 and N2O, would still likely be concentration driven for the bulk of ESMs, given the nascent field of interactive gas cycles in ESMs (Sanderson et al., 2023; Hajima et al., 2024).
We suggest categories that should be represented by a representative emission pathway, starting from the highest-emission category (Table 1):
-
TEWA: “the emission world avoided”, a category for a pathway with high or very high emissions.
-
NFA: “no further action”, a category for a pathway reflecting current emission futures in the absence of any further climate action. The pathways in this category should ideally be accompanied by a perturbed physics ensemble (acknowledging that those come with additional challenges of drifts, flux corrections, etc.; e.g. Shiogama et al., 2012) as this would allow us to obtain valuable proxies for a worst-case high-end warming outcome under emissions implied by current policies (low likelihood/high impact).
-
DASMT: “delayed climate action and stabilisation pathway missing target”, a category for a pathway that misses the Paris Agreement long-term temperature goal as it results in global warming of around 2 °C in 2100, rather than staying “well below” 2 °C. Such a pathway explores global emissions being approximately in line with NDCs and long-term targets as they were proposed around the time of the Glasgow Climate Pact, COP26 (Meinshausen et al., 2022; Rogelj et al., 2023).
-
DAPD: “delayed action peak and decline”, a category for a pathway in which climate action is further delayed but then features rapid emission declines and strongly negative long-term CO2 emissions.
-
IAPD: “immediate action peak and decline”, a category for a pathway that features immediate 2025 onset of decisive emission reductions and achieves net-zero CO2 emissions by mid-century.
-
IA2015: “immediate action in 2015”, a category for a pathway that resembles “a world that could have been” at the low-emission end, assuming that emission reductions towards net zero had started in 2015. Other “world that could have been” scenarios can be envisioned and could be policy relevant, e.g. one starting in 1992 with the establishment of the UNFCCC.
Table 1Overview of suggested pathway categories to inform the design of specific representative emission pathways for ESM simulations. Categories of emission pathways identified in IPCC AR6 WGIII (compare Table SPM.1 therein) and selected WGI core SSP–RCP scenarios are provided for comparison. For each category, we provide an indicative “priority” suggestion, recognising that there are limited resources to run a large set of scenarios across all ESMs.
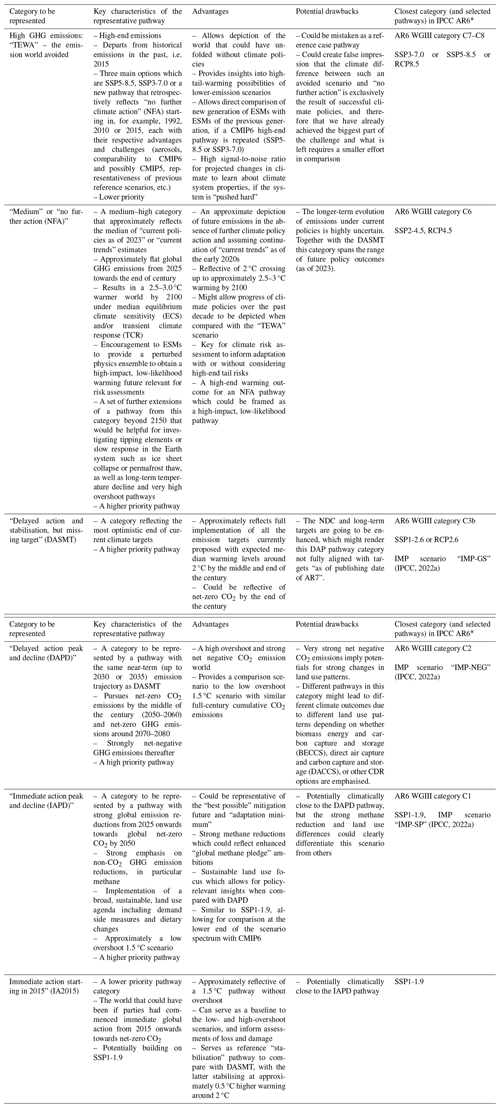
* No socioeconomic storylines should be associated with the next generation of pathways, similar to RCPs.
Focusing on largely emission-driven simulations while at the same time providing a framework that envisages certain global warming futures being investigated requires methodological clarification, as ESMs will produce different future global surface temperature outcomes for the same emission future. To a lesser degree, that same issue existed in the previous generations of RCP and SSP climate pathways, as ESMs did not share the same internal radiative forcing for the same input dataset of concentrations, emissions and land use patterns, yet they were all labelled 1.9 W m−2 or 2.6 W m−2 pathways. We propose two ways to address this issue: firstly, the headline names of the scenarios do not include the temperature level, but rather a qualitative label on the emission pathway. Secondly, we suggest continuing the practice of using the previous IPCC assessment cycle's findings (here, AR6) to design the scenarios for the next assessment cycle. For example, the SSP scenarios for the CMIP6 and the AR6 report, including the “Special Report on Global Warming of 1.5 °C”, were selected to match 1.9 W m−2 or 2.6 W m−2 labels by using a default AR5-calibrated MAGICC version, even though AR6 was expected to advance our knowledge on carbon cycle, other gas cycles and radiative efficiencies. Thus, an SSP1-2.6 pathway under the AR6 assessed science on gas cycles and radiative forcing will neither necessarily result in a 2.6 W m−2 median forcing nor will this forcing level be uniform across the ESMs. Similarly, but somewhat more pronouncedly, we suggest that the emission pathways be designed using the AR6-calibrated climate emulators to match the design criteria while at the same time acknowledging that the advancement of science will result in some shift in the best-estimate temperature projections (compared with what AR6-calibrated emulators produce).
We note that for some of the research questions identified, in particular in relation to overshoot and long-term (ir)reversibility, the pathway extensions beyond 2150 are of particular relevance. Stylised extensions for SSP–RCP scenarios have been provided until 2500 (Meinshausen et al., 2020) and we suggest considering this time frame also for extending the framing pathways. However, we would suggest moving beyond stylised extensions and explicitly considering the policy and research questions we outlined above in the design of the pathway extensions. In particular, it might be advisable to consider more than one extension per pathway, i.e. explore the effects of long-term temperature stabilisation vs. decline from the same emission pathway in 2150.
5.1 Framing pathway storylines consistent with recent IEA and NGFS scenarios
We note that the set of categories identified here – which are to be represented by specific framing pathways – bear some similarities to scenarios that have been identified by other initiatives, not necessarily related to the IPCC assessment, that are of key policy relevance, such as the scenario set investigated by the International Energy Agency (IEA) (IEA, 2022) and the Network for Greening the Financial Sector (NGFS) (Richters et al., 2022). In particular, those key pathway sets examine the worlds under current policies, current climate targets on the higher side and emission levels consistent with the ultimate goal of the Paris Agreement on the lower side. To us, this illustrates a convergence in views of what policy-relevant perspectives are on pathways that the climate science community may want to consider. The initial June 2023 workshop discussions for the forthcoming ScenarioMIP protocol, at which this paper's proposal was presented, also picks up some elements of this convergence of views (Fig. 1 in van Vuuren et al., 2023). We hope that the science and policy objectives, as well as the presented pathway categories and timing considerations, outlined in this paper can further inform the deliberations under ScenarioMIP, as well as other MIPs, under the CMIP umbrella.
5.2 High granularity of lower pathway categories
We have identified three climate pathway categories between 1.5 °C and “below 2 °C”. One of the pathway categories (IA2015), a lower priority one, represents a low “the world that could have been” pathway, i.e. it investigates a hypothetical world in which global emissions would have diverged from historical emissions in 2015 to stay below 1.5 °C. The other two categories, IAPD and DAPD (see Table 1 above), resemble the lowest two AR6 WGIII categories C1 and C2 that in the central outcome are expected to return global-mean temperatures to below 1.5 °C by the end of the century again. One argument against a high granularity of ESM simulations is often that they are too close to each other to detect climate differences. This presumption is in direct contrast with a high level message from the IPCC AR6 that “every bit of warming matters” and requires further reflection. Firstly, differences between small increments of warming can indeed be detected (e.g. Pfleiderer et al., 2018) including for long-term sea level rise where differences in peak warming of 0.2 °C may amount to a 40 cm difference in 2300 sea level rise commitment or more (Mengel et al., 2018), and also in the near-term emergence of climate extremes on the country level when considering large ensembles (Beusch et al., 2022a). While for a single ESM framework large ensembles of simulations are required to statistically investigate differences in “close by” scenarios or weak single-forcing differences (Shiogama et al., 2023; Smith et al., 2022), the multi-model nature of CMIP could allow us to investigate the multi-model average differences across close-by scenarios, without the need for each modelling centre to generate very large ensembles. Furthermore, while pathways might differ by less than 0.2 °C in terms of global-mean temperature, differences in regional emissions across those scenarios imply stronger differences in regional climate outcomes (e.g. Persad et al., 2023). In addition, the category characteristics outlined above would imply very different land use futures. These differences would translate to different climate futures in particular at the regional level and, given the differences in the representation of land–climate feedbacks across ESMs, also a range of different global climate outcomes that are of key importance to inform the policy discourse on land-based mitigation.
In addition to differences in peak warming, very different overshoot outcomes would be implied under an IAPD- in comparison with a DAPD-type future. A systematic exploration of those differences, and the robustness of different mitigation strategies when considering climate impacts, is critically important given the profound differences between such pathways in the mitigation space. Whether we advance mitigation efforts to achieve a low overshoot instead of a higher one is a question of high societal relevance. To meet the high policy interest around future temperature outcomes (that can be argued to be) within the long-term Paris Agreement goals, the AR6 WGIII report (IPCC, 2022a), for example, placed strong emphasis on so-called illustrative mitigation pathway (IMP) scenarios that are largely in the lower 1.5 °C with no or low overshoot category. Similarly, we argue that it is time to enable WG II to provide the corresponding impact assessment via adequate ESM simulations that provide the needed geophysical input for ecosystem, land use and biogeophysical impact models, as well as associated impact studies. Those types of models that directly feed off ESM output have emerging importance in the quest to arrive at a finer-grained picture of future impacts and their difference between different pathways.
Lastly, sometimes the argument is made that emerging pattern scaling or regional emulator approaches could fill in and extrapolate ESM results. While that might be increasingly possible in the future (especially if proposed ESM experiments provide good training datasets), the current set of regional emulators is not yet able to fill that niche. That is particularly true for peak-and-decline (overshoot) scenarios that have the potential to exhibit hysteresis in terms of large-scale and regional warming and precipitation patterns (Pfleiderer et al., 2023). For example, approaches that rely on global-mean temperatures as one of their input parameters are not yet adequately capable of distinguishing warming, stable and cooling worlds, before, at or after a peak in global-mean temperatures (see point 5.6 below).
An additional pathway that could be similar to the delayed action DAPD one could be one that limits peak warming to below 2 °C with a likely (66 %) chance and also avoids strongly negative emissions. It would miss the 1.5 °C warming level by 2100 and arguably not be in line with the Paris Agreement's “pursuing efforts for 1.5 °C” element, and most probably also not with achieving net-zero greenhouse gases, but could present a third plausible pathway within the 1.5 to below 2 °C range (similarly to “C3” category pathways investigated in IPCC AR6 WGIII). Given the overall resource constraints, we consider the pair of IAPD and DAPD pathways to provide more relevant scientific and policy-relevant insights compared with either an IAPD–C3 or a DAPD–C3 combination, yet we acknowledge the advantage that a triple IAPD, DAPD and C3 investigation might bring. The reason is that only DAPD would explore a strong overshoot and net negative emission behaviour, and without IAPD it would be impossible to adequately illustrate the lower region of plausible futures.
In summary, we suggest that future framing pathways separately explore pathways from an immediate-action, sustainable-future category (IAPD) and a delayed-action, high-overshoot, high-negative-emission category (DAPD). These categories could arguably also be seen as exploring different futures within the Paris Agreement temperature goal range. Having at least two scenarios will prevent a singular defacto definition by the scientific community of what the Paris Agreement goal means exactly (which could be regarded as policy prescriptive). Exploring pathway variants that yield different pathways within the Paris Agreement temperature goal range obviously belongs to one of the most highly policy-relevant questions (IPCC, 2023) and should be a more than worthwhile investment of resources.
5.3 High warming pathway
Our deliberations still include a high warming pathway category, i.e. “the emission world avoided”, as those high warming pathways are widely used in the community and serve the scientific purpose of understanding climate change under large forcings. In addition, the more idealised 1 % CO2 and abrupt forcing runs adequately assist the scientific quest to better understand Earth system characteristics in a high forcing/high warming world. Several scientific applications, i.e. related to emulator calibration and global warming level assessments, would also continue to rely on such high forcing pathways, and we do not argue that such high forcing outcomes should not be modelled in the next generation of ESM framing pathways. Our proposal, however, no longer includes a high warming pathway category that could be mistaken for a “business-as-usual” scenario and we argue that it would be beneficial to separate high forcing pathways for scientific purposes from the more policy-oriented framing pathway categories.
The high-end “emission world avoided” pathway at the upper end could also serve a strong communication purpose. Frequently, the success of the Montreal Protocol in limiting the emissions of ozone-depleting substances is showcased by comparing current emissions to “the world avoided” scenarios (Velders et al., 2007). Having a similar comparison point or range in climate science would be a useful indicator of where we might have been if we had failed to put climate action on the political agenda. In contrast, at the other end, exploring the low-end emissions “world that might have been” is also a reminder of what we could have achieved if not for political and economic forces that inhibited swift global-scale emission reductions over the past decades (Supran et al., 2023).
5.4 Separate consideration of socioeconomic pathways
It is worth noting that this proposed set of scenarios does not prescribe specific socioeconomic futures but can accommodate different narratives of socioeconomic development as reflected in the original shared socioeconomic pathway (SSP) framework, as well as different perspectives on burden sharing, equity and fairness that have been identified as key elements of scenario development arising from the Bangkok discussions. The one methodological challenge will be to derive characteristic land use and aerosol precursor emission patterns that are representative of some future socioeconomic evolutions, with potential variations then studied in additional sensitivity MIPs (Sect. 5.5).
5.5 Framing pathways to be complemented by sensitivity MIPs
A limited set of climate “framing pathways” should be complemented by a much broader set of explorations in different MIPs under the new round of CMIP. Those sensitivity cases should explore land use patterns, different aerosol assumptions (both spatially and different time series evolutions), methane and other ozone precursor reductions, global warming levels, etc. However, in our view the climate “framing pathways” presented here would provide a good basis for interlinkages with different MIP explorations on those key topics and key research questions as outlined above. We also note other, potentially policy-relevant emerging approaches for scenario design beyond emission- or concentration-driven simulations that explore adaptive, ESM-specific approaches to match different warming level outcomes, thus filling a critical gap in explicitly exploring warming level dependent impacts in the ESM space (Terhaar et al., 2022).
5.6 Regionally explicit emulators are still in the early stages of development
Separate from regional downscaling that benefits from a long tradition and uses ESM output as a starting point, the question has emerged as to what degree regionally explicit emulators can replace ESM simulations. As mentioned in Sect. 5.2, the use of emerging tools, such as spatially explicit emulators in combination with well-established reduced-complexity climate models (e.g. Beusch et al., 2020, 2022b; Tebaldi et al., 2022), can provide for very promising applications to explore a range of different futures in rapid fashion. However, such approaches, often based on the idea of pattern scaling of global-mean temperature, can only be used for emulation of ESM output, and without adequate ESM training pathways, they will not be able to capture key features of peak-and-decline or stabilisation pathways, for which the central assumption of scaling with global-mean temperatures does not hold anymore in the same way (Pfleiderer et al., 2023). Furthermore, none of those emulators are yet able to provide the full richness of variables, as well as the spatial, temporal and cross-variable correlations that ESMs are able to provide and which are key for a range of specific impact models such as those linked in the ISIMIP project (Frieler et al., 2023; Warszawski et al., 2014). Thus, while emulators will be able to play an increasingly important role, they do not provide sufficient capabilities to address the science and policy questions identified above. ESMs therefore remain the key tool to synthesise, diagnose and analyse our best available climate system science under various driving forces. This might change at some point, but it would be a large risk to bet that emulators will be sufficiently mature to fill our key scenario gaps by the time of AR7.
5.7 Linking projections of GHG and ozone-depleting substances, as well as new gases
To date, projections of the effects of atmospheric accumulation of GHGs, ozone-depleting substances (ODSs) and ODS replacements (hydrofluorocarbons (HFCs)) have occurred primarily through two communities: the IPCC for GHGs and the WMO/UNEP Ozone Depletion Assessments (e.g. World Meteorological Organization, 2022; Velders and Daniel, 2014) for ODSs and HFCs. While there has been increasing connectivity and collaboration between these two communities, projections of GHG as well as emissions of ODSs and their replacements have often been generated through substantially independent frameworks resulting in the underlying emission “storylines” often not being consistent. For example, in a given ozone-depletion assessment, the baseline ODS scenario from the previous assessment has been commonly used as the baseline for the 3-D model calculations. This can lead to inconsistencies when IPCC assesses and uses a new set of ODS emission scenarios to quantify the direct and indirect radiative forcings of ODSs and their replacements, with these scenarios often being distinctly different from any WMO/UNEP ODS scenarios. Furthermore, even the framework scenarios assessed by the IPCC scenarios, whether to attain an overall radiative forcing by some year or to follow a prescribed global development path, is not consistent with the approach taken by WMO/UNEP assessments. Given this and given that ESMs now increasingly include an interactive stratospheric chemistry scheme that explicitly couples the chemistry–climate effects of atmospheric GHG and ODS loading, a set of self-consistent GHG and ODS emission scenarios would be another opportunity to link these two research communities and provide a single set of simulations that can serve the needs of both communities, as well as benefit their intended policymaker stakeholders. As industrial processes, energy generation and transport activities transition to GHG and ODS alternatives, it is essential that the next generation of REP climate pathways include the new compounds emitted from the associated activities (e.g. the effects of fugitive hydrogen emissions on atmospheric chemistry, the stratosphere and climate from a hydrogen-based economy (e.g. Tromp et al., 2003)).
5.8 Timely provision of updated pathway information to inform the global stocktake
Countries striving to implement their climate plans, including mitigation and adaptation objectives as well as responses to loss and damage, will require significant investments in the envisioned economic and societal transformation (IPCC, 2022a). To inform policy and investment decisions, timely provision of scientific analysis and assessments is key. In response to this demand, agencies such as the IEA or the NGFS provide annual updates of emission pathways. Such a rigid timeline is impractical for community pathway developments focused on ESMs. However, we would argue that a reflection on the timeline for pathway design in the light of stakeholder needs, and in particular political processes under the Paris Agreement, is very much in order.
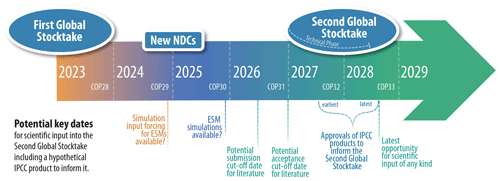
Figure 3A stylised timeline for scientific information and IPCC to inform the second global stocktake. Key dates are derived based on the global stocktake cycle and its modalities, including a technical phase for scientific input. The stylised timeline for IPCC products allows the establishment of a schedule for the design and execution of a new generation of ESM simulations aimed at informing the relevant IPCC products. Note that cut-off dates are indicated relative to the earliest IPCC product approval timing and would move accordingly backwards for a later approval date.
The second global stocktake (GST) under the Paris Agreement will be conducted in 2027/2028 (Fig. 3). Informed by its outcomes, parties to the agreement will be invited to develop their NDCs for 2040. A 2040 horizon is close to expressed net-zero CO2 and net-zero GHG targets for several major emitters, and very deep emission reductions, as well as building up carbon dioxide removal capacities, will be required to achieve them (Edenhofer et al., 2023). Several of the policy and science questions identified above are directly relevant to informing the development of NDCs on this timeline. Striving to provide major scientific community inputs in time for the second GST will be essential. Thus, an enhanced timeline of some CMIP7 outputs (or also potential continued CMIP6 activities to fill critical gaps in the existing scenario space) is almost certainly required (CMIP Panel, 2024). It would be unfortunate if the best available science at the time of the second GST in 2027/2028 would still need to predominantly rely on a set of ESM scenarios dating back to 2015 (the current CMIP6 SSP–RCP framework).
Reflections on scientific inputs into the second GST need to be cognisant of the timeline of the IPCC 7th Assessment Report (AR7) cycle, as the IPCC will be a key source of input to the global stocktake process. Even before the AR7 had started with the election of its new Bureau in July 2023, the discussions at the Bangkok meeting suggested the need to consider producing relevant outputs by 2028 (IPCC, 2023). As part of the COP28 decisions on the outcome of the first global stocktake and the IPCC AR6, the UNFCCC invited the IPCC to “consider how best to align its work with the second and subsequent global stocktakes” and “to provide relevant and timely information for the next global stocktake” (1/CMA.5, paragraph 184 in UNFCCC, 2023b), and also invited the IPCC to “continue providing relevant information to Parties on the scientific, technical and socioeconomic aspects of climate change and to take into account, in determining its future products and assessment cycles, work under the Convention and the Paris Agreement” (see 20/CMA.5 paragraph iv in UNFCCC, 2023a). At its 60th session in January 2024, the IPCC considered the above invitations and decided that the AR7 work programme will include the three Working Group Assessment reports, a Synthesis Report, the previously agreed “Special Report on Climate Change and Cities” and two TFI Methodology reports on short-lived climate forcers and carbon dioxide removal technologies, carbon capture utilisation and storage. Since no additional dedicated special report was agreed, the three IPCC Working Group Assessment reports will provide the only avenue to adequately inform the second GST. Figure 3 depicts the time frame of when this series of IPCC products will need to be produced given past experience and the hard deadline presented by the second GST. From that range, we can work backwards with potential literature cut-off dates for any such product. Based on this, we argue that aiming for providing ESM data by mid to end 2025, and thereby making forcing data available by late 2024, would be required. This tight timeline highlights the need for early preparation and reflection by the scientific community well ahead of the finalisation of the IPCC schedule. Furthermore, the challenges experienced in AR6 with “hot models” might caution against making simulations available too close to the deadline (Hausfather et al., 2022).
Missing the opportunity for input into the second GST will not only just forego the chance to inform one of the key processes for scientific input under the UNFCCC, but, and arguably even more importantly, also the time window where countries can still take on board new climate science insights in the preparation of their NDCs with a 2040 target year. Furthermore, some link between the IPCC timelines and the international climate change deliberations between countries seems pertinent to fulfil the IPCC's mandate to be policy relevant, as well as necessary to maintain and build the IPCC's standing in the international discourse.
A myriad of factors inform scenario design processes for the next generation of ESMs. For the selection of framing pathways for coordinated ESM simulations (e.g. the SSP–RCPs in CMIP6) in particular we believe that policy considerations need to be fully taken into account given their relevance for the IPCC assessment, and to inform climate policy and action more generally. One key challenge is to not constrain the future climate space in a manner that excludes exploring expressed policy objectives and hence might prevent forthcoming IPCC assessments from fulfilling their mandate to provide a policy-relevant, yet not policy-prescriptive, assessment (IPCC, 2023). Given that ongoing scenario design processes under the CMIP umbrella will effectively set the framing scenarios on which any forthcoming IPCC assessment will be based, considerations on how to avoid potential policy-prescriptive choices by the research community need to be considered very carefully, considering a comprehensive range of policy outcomes that include representation of the maximum ambition of the Paris Agreement (limiting warming to 1.5 °C) and potential high-end outcomes.
When it comes to the representation of pathways that could be considered Paris Agreement compatible, it is important for science to be ready and capable of providing an open, transparent and full appraisal of the co-benefits, efforts and changes from past practices that might be warranted in order to pursue certain warming outcomes. Focus on the lower range of scenarios is also reflective of a post-Paris policy landscape in which questions are no longer about whether or not climate targets will be set, but about the credibility, risk and implementation of proposed pathways to meet those targets as well as defining the necessary pace of climate action required to achieve them (Meinshausen et al., 2022; Rogelj et al., 2023). Informing such reflections also requires scientific information on the consequences of not achieving certain collective policy ambitions and process-based assessments of the implicit technological and physical assumptions in those pathways.
This progression in the climate discourse is also informed by one of the main messages from the IPCC's AR6 report series, that “every increment of global warming” matters. Further substantiating such an assessment will be critical to climate science and forthcoming IPCC assessments, which require new framing pathways to provide sufficiently granular resolution, not just for end-of-century (or beyond) global warming outcomes, but even more so on the decision-making relevant horizon until mid-century.
As we argue in this paper, science and policy questions for the next generation of framing pathways also include those related to a potential temperature overshoot and return, as well as the benefits and negative side effects – along with the technological and physical plausibility – of large-scale carbon dioxide removal. We further argue that due consideration should be given to how a community effort for the development of new scenarios and related scientific insights can inform the second global stocktake under the Paris Agreement and the development of new submissions of NDCs for the target year 2040.
Based on these considerations, we have identified a range of categories that would, together, satisfy key considerations and could be represented by framing pathways called representative emission pathways (REPs). Considerations beyond those outlined here may inform the ongoing efforts of pathway design as part of CMIP, including ScenarioMIP (van Vuuren et al., 2023) and/or other model intercomparison projects. We hope that our perspective can serve as input to a strategic approach for driving ESMs with policy-relevant futures and contribute to further community reflections on the next generation of framing pathways that we think will greatly benefit from an open and inclusive discussion given the far-reaching consequences for climate science and policy stemming from scenario design.
No data has been generated and figures are illustrative only.
MM wrote the first draft and CFS edited the first draft based on discussions at the IPCC Expert Workshop in Bangkok, April 2023. Further development was co-led by MM and CFS. GPP and ZN provided a first sketch of the conceptual overview, Fig. 1, implemented by MM and CFS. All authors contributed to the paper.
The contact author has declared that none of the authors has any competing interests.
Publisher's note: Copernicus Publications remains neutral with regard to jurisdictional claims made in the text, published maps, institutional affiliations, or any other geographical representation in this paper. While Copernicus Publications makes every effort to include appropriate place names, the final responsibility lies with the authors.
The authors thank Matthew Gidden, Alaa Al Khourdajie, Hideo Shiogama, Janna Sillmann, Anna Zehrung and other valued colleagues for very helpful comments on an earlier draft. Carl-Friedrich Schleussner, Benjamin M. Sanderson, Adelle Thomas, Sophie Szopa and Joeri Rogeli acknowledge funding from the European Union's Horizon 2020 research and innovation programmes under grant agreement no. 101003687 (PROVIDE). Glen P. Peters acknowledges funding from the European Union's Horizon 2020 research and innovation programmes under grant agreement no. 821003 (4C) and the European Union's Horizon Europe Research and Innovation Programme under grant agreement no. 101056306 (IAM COMPACT). Roland Séférian and Zebedee Nicholls acknowledge funding from the European Union's Horizon 2020 research and innovation programmes under grant agreement no. 101003536 (ESM2025). Malte Meinshausen, Andrew D. King, Josep G. Canadell and Tilo Ziehn acknowledge funding from the Australian National Environmental Science Program – Climate Systems Hub.
This research has been supported by the EU Horizon 2020 programme (grant nos. 101003687, 821003, 101056306 and 101003536) and the Australian National Environmental Science Program – Climate Systems Hub.
This open-access publication was funded by the Humboldt-Universität zu Berlin.
This paper was edited by Gunnar Luderer and David Ham and reviewed by Andy Reisinger and Dale Rothman.
Asaadi, A., Schwinger, J., Lee, H., Tjiputra, J., Arora, V., Séférian, R., Liddicoat, S., Hajima, T., Santana-Falcón, Y., and Jones, C. D.: Carbon cycle feedbacks in an idealized simulation and a scenario simulation of negative emissions in CMIP6 Earth system models, Biogeosciences, 21, 411–435, https://doi.org/10.5194/bg-21-411-2024, 2024.
Beusch, L., Gudmundsson, L., and Seneviratne, S. I.: Emulating Earth system model temperatures with MESMER: from global mean temperature trajectories to grid-point-level realizations on land, Earth Syst. Dynam., 11, 139–159, https://doi.org/10.5194/esd-11-139-2020, 2020.
Beusch, L., Nauels, A., Gudmundsson, L., Gütschow, J., Schleussner, C.-F., and Seneviratne, S. I.: Responsibility of major emitters for country-level warming and extreme hot years, Commu. Earth Environ., 3, 7, https://doi.org/10.1038/s43247-021-00320-6, 2022a.
Beusch, L., Nicholls, Z., Gudmundsson, L., Hauser, M., Meinshausen, M., and Seneviratne, S. I.: From emission scenarios to spatially resolved projections with a chain of computationally efficient emulators: coupling of MAGICC (v7.5.1) and MESMER (v0.8.3), Geosci. Model Dev., 15, 2085–2103, https://doi.org/10.5194/gmd-15-2085-2022, 2022b.
Brunner, L., Pendergrass, A. G., Lehner, F., Merrifield, A. L., Lorenz, R., and Knutti, R.: Reduced global warming from CMIP6 projections when weighting models by performance and independence, Earth Syst. Dynam., 11, 995–1012, https://doi.org/10.5194/esd-11-995-2020, 2020.
Canadell, J. G., Meyer, C. P., Cook, G. D., Dowdy, A., Briggs, P. R., Knauer, J., Pepler, A., and Haverd, V.: Multi-decadal increase of forest burned area in Australia is linked to climate change, Nat. Commun., 12, 6921, https://doi.org/10.1038/s41467-021-27225-4, 2021.
Ciavarella, A., Stott, P., and Lowe, J.: Early benefits of mitigation in risk of regional climate extremes, Nat. Clim. Change, 7, 326–330, https://doi.org/10.1038/nclimate3259, 2017.
CMIP Panel: AR7 Fast Track community consultation, https://wcrp-cmip.org/ar7-fast-track-community-consultation/, last access: 14 March 2024.
Edenhofer, O., Jacobsen, J. B., Anadón, L. D., van Aalst, M., Cartalis, C., Dessai, S., Eory, V., Hertwich, E., Kitzing, L., López-Gunn, E., Nilsson, L. J., Riahi, K., Rogelj, J., Schrijver, N., and Soussana, J.-F.: Scientific advice for the determination of an EU-wide 2040 climate target and a greenhouse gas budget for 2030–2050, European Union, Brussels, https://climate-advisory-board.europa.eu/reports-and-publications/scientific-advice-for-the-determination-of-an-eu-wide-2040 (last access: 3 May 2024), 2023.
ENB: Earth Negotiation Bulletin – Summary Report, 13–19 March 2023, 58th Session of the IPCC (IPCC-58), IISD, https://enb.iisd.org/58th-session-intergovernmental-panel-climate-change-ipcc-58-summary (last access: 3 May 2024), 2023.
Eyring, V., Bony, S., Meehl, G. A., Senior, C. A., Stevens, B., Stouffer, R. J., and Taylor, K. E.: Overview of the Coupled Model Intercomparison Project Phase 6 (CMIP6) experimental design and organization, Geosci. Model Dev., 9, 1937–1958, https://doi.org/10.5194/gmd-9-1937-2016, 2016.
Folberth, G. A., Staniaszek, Z., Archibald, A. T., Gedney, N., Griffiths, P. T., Jones, C. D., O'Connor, F. M., Parker, R. J., Sellar, A. A., and Wiltshire, A.: Description and Evaluation of an Emission-Driven and Fully Coupled Methane Cycle in UKESM1, J. Adv. Model. Earth Sy., 14, e2021MS002982, https://doi.org/10.1029/2021MS002982, 2022.
Forster, P., Storelvmo, T., Armour, K., Collins, W., Dufresne, J.-L., Frame, D., Lunt, D. J., Mauritsen, T., Palmer, M. D., Watanabe, M., Wild, M., and Zhang, H.: The Earth's Energy Budget, Climate Feedbacks, and Climate Sensitivity, in: Climate Change 2021: The Physical Science Basis, Contribution of Working Group I to the Sixth Assessment Report of the Intergovernmental Panel on Climate Change edited by: Masson-Delmotte, V., Zhai, P., Pirani, A., Connors, S. L., Péan, C., Berger, S., Caud, N., Chen, Y., Goldfarb, L., Gomis, M. I., Huang, M., Leitzell, K., Lonnoy, E., Matthews, J. B. R., Maycock, T. K., Waterfield, T., Yelekçi, O., Yu, R., and Zhou, B., Cambridge University Press, Cambridge, UK and New York, USA, 923–1054, 2021.
Frieler, K., Volkholz, J., Lange, S., Schewe, J., Mengel, M., del Rocío Rivas López, M., Otto, C., Reyer, C. P. O., Karger, D. N., Malle, J. T., Treu, S., Menz, C., Blanchard, J. L., Harrison, C. S., Petrik, C. M., Eddy, T. D., Ortega-Cisneros, K., Novaglio, C., Rousseau, Y., Watson, R. A., Stock, C., Liu, X., Heneghan, R., Tittensor, D., Maury, O., Büchner, M., Vogt, T., Wang, T., Sun, F., Sauer, I. J., Koch, J., Vanderkelen, I., Jägermeyr, J., Müller, C., Rabin, S., Klar, J., Vega del Valle, I. D., Lasslop, G., Chadburn, S., Burke, E., Gallego-Sala, A., Smith, N., Chang, J., Hantson, S., Burton, C., Gädeke, A., Li, F., Gosling, S. N., Müller Schmied, H., Hattermann, F., Wang, J., Yao, F., Hickler, T., Marcé, R., Pierson, D., Thiery, W., Mercado-Bettín, D., Ladwig, R., Ayala-Zamora, A. I., Forrest, M., and Bechtold, M.: Scenario setup and forcing data for impact model evaluation and impact attribution within the third round of the Inter-Sectoral Impact Model Intercomparison Project (ISIMIP3a), Geosci. Model Dev., 17, 1–51, https://doi.org/10.5194/gmd-17-1-2024, 2024.
Frölicher, T. L. and Joos, F.: Reversible and irreversible impacts of greenhouse gas emissions in multi-century projections with the NCAR global coupled carbon cycle-climate model, Clim. Dynam., 35, 1439–1459, 2010.
Fuss, S., Lamb, W. F., Callaghan, M. W., Hilaire, J., Creutzig, F., Amann, T., Beringer, T., de Oliveira Garcia, W., Hartmann, J., Khanna, T., Luderer, G., Nemet, G. F., Rogelj, J., Smith, P., Vicente, J. L. V., Wilcox, J., del Mar Zamora Dominguez, M., and Minx, J. C.: Negative emissions – Part 2: Costs, potentials and side effects, Environ. Res. Lett., 13, 063002, https://doi.org/10.1088/1748-9326/aabf9f, 2018.
Gasser, T., Kechiar, M., Ciais, P., Burke, E. J., Kleinen, T., Zhu, D., Huang, Y., Ekici, A., and Obersteiner, M.: Path-dependent reductions in CO2 emission budgets caused by permafrost carbon release, Nat. Geosci., 11, 830–835, https://doi.org/10.1038/s41561-018-0227-0, 2018.
Gidden, M. J., Riahi, K., Smith, S. J., Fujimori, S., Luderer, G., Kriegler, E., van Vuuren, D. P., van den Berg, M., Feng, L., Klein, D., Calvin, K., Doelman, J. C., Frank, S., Fricko, O., Harmsen, M., Hasegawa, T., Havlik, P., Hilaire, J., Hoesly, R., Horing, J., Popp, A., Stehfest, E., and Takahashi, K.: Global emissions pathways under different socioeconomic scenarios for use in CMIP6: a dataset of harmonized emissions trajectories through the end of the century, Geosci. Model Dev., 12, 1443–1475, https://doi.org/10.5194/gmd-12-1443-2019, 2019.
Hajima, T., Kawamiya, M., Ito, A., Tachiiri, K., Jones, C., Arora, V., Brovkin, V., Séférian, R., Liddicoat, S., Friedlingstein, P., and Shevliakova, E.: Consistency of global carbon budget between concentration- and emission-driven historical experiments simulated by CMIP6 Earth system models and suggestion for improved simulation of CO2 concentration, EGUsphere [preprint], https://doi.org/10.5194/egusphere-2024-188, 2024.
Hausfather, Z. and Peters, G. P.: Emissions–the “business as usual” story is misleading, Nature, 577, 618–620, 2020.
Hausfather, Z., Marvel, K., Schmidt, G. A., Nielsen-Gammon, J. W., and Zelinka, M.: Climate simulations: Recognize the “hot model” problem, Nature, 605, 26–29, 2022.
Hoegh-Guldberg, O., Jacob, D., Taylor, M., Bindi, M., Brown, S., Camilloni, I., Diedhiou, A., Djalante, R., Ebi, K. L., Engelbrecht, F., Guiot, J., Hijioka, Y., Mehrotra, S., Payne, A., Seneviratne, S. I., Thomas, A., Warren, R., and Zhou, G.: Impacts of 1.5 °C Global Warming on Natural and Human Systems, in: Global Warming of 1.5 °C. An IPCC Special Report on the impacts of global warming of 1.5 °C above pre-industrial levels and related global greenhouse gas emission pathways, in the context of strengthening the global response to the threat of climate change, sustainable development, and efforts to eradicate poverty, edited by: IPCC, Cambridge University Press, Cambridge, UK and New York, NY, USA, 175–312, https://doi.org/10.1017/9781009157940.005, 2018.
Huard, D., Fyke, J., Capellán-Pérez, I., Matthews, H. D., and Partanen, A.-I.: Estimating the Likelihood of GHG Concentration Scenarios From Probabilistic Integrated Assessment Model Simulations, Earth's Future, 10, e2022EF002715, https://doi.org/10.1029/2022EF002715, 2022.
Humpenöder, F., Popp, A., Schleussner, C.-F., Orlov, A., Windisch, M. G., Menke, I., Pongratz, J., Havermann, F., Thiery, W., Luo, F., v. Jeetze, P., Dietrich, J. P., Lotze-Campen, H., Weindl, I., and Lejeune, Q.: Overcoming global inequality is critical for land-based mitigation in line with the Paris Agreement, Nat. Commun., 13, 7453, https://doi.org/10.1038/s41467-022-35114-7, 2022.
IEA: World Energy Outlook 2022, IEA, Paris, France, https://www.iea.org/reports/world-energy-outlook-2022 (last access: 3 May 2024), 2022.
IPCC: Towards New Scenarios for Analysis of Emissions: Climate Change, Impacts, and Response Strategies, edited by: Moss, R., Babiker, M., Brinkman, S., Calvo, E., Carter, T., Edmonds, J., Elgizouli, I., Emori, S., Erda, L., and Hibbard, K., Intergovernmental Panel on Climate Change Geneva, Switzlerland, 132 pp., https://archive.ipcc.ch/pdf/supporting-material/expert-meeting-report-scenarios.pdf (last access: 3 May 2024), 2008.
IPCC: Meeting Report of the Intergovernmental Panel on Climate Change Expert Meeting on Scenarios, edited by: Riahi, K., Minx, J. C., Barros, V., Bustamente, M., Carter, T., Edenhofer, O., Field, C., Kriegler, E., Lamarque, J.-F., Mach, K., Mathur, R., O'Neill, B., Pichs-Madruga, R., Plattner, G.-K., Qin, D., Sokona, Y., Stocker, T., Zhou, T., Antle, J., Arnell, N., Edmonds, J., Emori, S., Friedlingstein, P., Fuglestvedt, J., Joos, F., Lotze-Campen, H., Lu, X., Meinshausen, M., Nakicenovic, N., Prather, M., Preston, B., Rao, N., Rogelj, J., Rozenberg, J., Shukla, P. R., Skea, J., Tebaldi, C., and Vuuren, D. V., Potsdam Institute for Climate Impact Research, Potsdam, Germany, 57 pp., https://www.ipcc.ch/site/assets/uploads/2018/05/EMR_Scenarios-1.pdf (last access: 3 May 2024), 2016.
IPCC: Global Warming of 1.5 °C. An IPCC Special Report on the impacts of global warming of 1.5 °C above pre-industrial levels and related global greenhouse gas emission pathways, in the context of strengthening the global response to the threat of climate change, sustainable development, and efforts to eradicate poverty, edited by: Masson-Delmotte, V., Zhai, P., Pörtner, H.-O., Roberts, D., Skea, J., Shukla, P. R., Pirani, A., Moufouma-Okia, W., Péan, C., Pidcock, R., Connors, S., Matthews, J. B. R., Chen, Y., Zhou, X., Gomis, M. I., Lonnoy, E., Maycock, T., Tignor, M., and Waterfield, T., Cambridge University Press, Cambridge, UK and New York, NY, USA, 3–24, https://doi.org/10.1017/9781009157940, 2018.
IPCC: Climate Change 2021: The Physical Science Basis, Contribution of Working Group I to the Sixth Assessment Report of the Intergovernmental Panel on Climate Change, edited by: Masson-Delmotte, V., Zhai, P., Pirani, A., Connors, S. L., Péan, C., Berger, S., Caud, N., Chen, Y., Goldfarb, L., Gomis, M. I., Huang, M., Leitzell, K., Lonnoy, E., Matthews, J. B. R., Maycock, T. K., Waterfield, T., Yelekçi, O., Yu, R., and Zhou, B., Cambridge University Press, https://doi.org/10.1017/9781009157896, 2021.
IPCC: Climate Change 2022: Mitigation of Climate Change. Contribution of Working Group III to the Sixth Assessment Report of the Intergovernmental Panel on Climate Change, edited by: Shukla, P. R., Skea, J., Slade, R., Al Khourdajie, A., van Diemen, R., McCollum, D., Pathak, M., Some, S., Vyas, P., Fradera, R., Belkacemi, M., Hasija, A., Lisboa, G., Luz, S., and Malley, J., Cambridge University Press, Cambridge University Press, Cambridge, UK and New York, NY, USA, https://www.ipcc.ch/report/ar6/wg3/ (last access: 3 May 2024), 2022a.
IPCC: Climate Change 2022: Impacts, Adaptation and Vulnerability. Contribution of Working Group II to the Sixth Assessment Report of the Intergovernmental Panel on Climate Change, Climate Change 2022: Impacts, Adaptation and Vulnerability. Contribution of Working Group II to the Sixth Assessment Report of the Intergovernmental Panel on Climate Change, edited by: Pörtner, H. O., Roberts, D. C., Tignor, M., Poloczanska, E. S., Mintenbeck, K., Alegría, A., Craig, M., Langsdorf, S., Löschke, S., Möller, V., Okem, A., and Rama, B., Cambridge University Press, Cambridge, UK and New York, NY, USA, 3056 pp., https://www.ipcc.ch/report/ar6/wg2/ (last access: 3 May 2024), 2022b.
IPCC: Workshop Report of the Intergovernmental Panel on Climate Change Workshop on the Use of Scenarios in the Sixth Assessment Report and Subsequent Assessments, edited by: Masson-Delmotte, V., Pörtner, H.-O., Roberts, D. C., Shukla, P. R., Skea, J., Zhai, P., Cheung, W., Fuglestvedt, J., Garg, A., O'Neill, B., Pereira, J., Portugal Pereira, J., Riahi, K., Sörensson, A., Tebaldi, C., Totin, E., van Vuuren, D., Zommers, Z., Al Khourdajie, A., Connors, S. L., Fradera, R., Ludden, C., McCollum, D., Mintenbeck, K., Pathak, M., Pirani, A., Poloczanska, E. S., Some, S., and Tignor, M., Working Group III Technical Support Unit, Imperial College London, United Kingdom, 67 pp., https://www.ipcc.ch/event/ipcc-workshop-on-the-use-of-scenarios-in-the-sixth-assessment-report-and-subsequent-assessments/ (last access: 3 May 2024), 2023.
Jones, C. D., Frölicher, T. L., Koven, C., MacDougall, A. H., Matthews, H. D., Zickfeld, K., Rogelj, J., Tokarska, K. B., Gillett, N. P., Ilyina, T., Meinshausen, M., Mengis, N., Séférian, R., Eby, M., and Burger, F. A.: The Zero Emissions Commitment Model Intercomparison Project (ZECMIP) contribution to C4MIP: quantifying committed climate changes following zero carbon emissions, Geosci. Model Dev., 12, 4375–4385, https://doi.org/10.5194/gmd-12-4375-2019, 2019.
Kanitkar, T., Mythri, A., and Jayaraman, T.: Equity assessment of global mitigation pathways in the IPCC Sixth Assessment Report, Climate Policy, 1–20, https://doi.org/10.1080/14693062.2024.2319029, 2024.
Kemp, L., Xu, C., Depledge, J., Ebi, K. L., Gibbins, G., Kohler, T. A., Rockström, J., Scheffer, M., Schellnhuber, H. J., and Steffen, W.: Climate Endgame: Exploring catastrophic climate change scenarios, P. Natl. Acad. Sci. USA, 119, e2108146119, https://doi.org/10.1073/pnas.2108146119, 2022.
King, A. D., Sniderman, J. K., Dittus, A. J., Brown, J. R., Hawkins, E., and Ziehn, T.: Studying climate stabilization at Paris Agreement levels, Nat. Clim. Change, 11, 1010–1013, 2021.
Kleinen, T., Gromov, S., Steil, B., and Brovkin, V.: Atmospheric methane underestimated in future climate projections, Environ. Res. Lett., 16, 094006, https://doi.org/10.1088/1748-9326/ac1814, 2021.
Koven, C. D., Arora, V. K., Cadule, P., Fisher, R. A., Jones, C. D., Lawrence, D. M., Lewis, J., Lindsay, K., Mathesius, S., Meinshausen, M., Mills, M., Nicholls, Z., Sanderson, B. M., Séférian, R., Swart, N. C., Wieder, W. R., and Zickfeld, K.: Multi-century dynamics of the climate and carbon cycle under both high and net negative emissions scenarios, Earth Syst. Dynam., 13, 885–909, https://doi.org/10.5194/esd-13-885-2022, 2022.
Kriegler, E., Edmonds, J., Hallegatte, S., Ebi, K. L., Kram, T., Riahi, K., Winkler, H., and van Vuuren, D. P.: A new scenario framework for climate change research: the concept of shared climate policy assumptions, Climatic Change, 122, 401–414, https://doi.org/10.1007/s10584-013-0971-5, 2014.
Lanson, A., Pfleiderer, P., Lehner, F., and Schleussner, C.-F.: Uncertainty in near-term temperature evolution must not obscure assessments of climate mitigation benefits, Nat. Commun., 13, 4029, https://doi.org/10.1038/s41467-022-31425-x, 2022.
Lee, J. Y., Marotzke, J., Bala, G., Cao, L., Corti, S., Dunne, J. P., Engelbrecht, F., Fischer, E., Fyfe, J. C., Jones, C., Maycock, A., Mutemi, J., Ndiaye, O., Panickal, S., and Zhou, T.: Future Global Climate: Scenario-Based Projections and Near-Term Information, in: Climate Change 2021: The Physical Science Basis, Contribution of Working Group I to the Sixth Assessment Report of the Intergovernmental Panel on Climate Change, edited by: Masson-Delmotte, V., Zhai, P., Pirani, A., Connors, S. L., Péan, C., Berger, S., Caud, N., Chen, Y., Goldfarb, L., Gomis, M. I., Huang, M., Leitzell, K., Lonnoy, E., Matthews, J. B. R., Maycock, T. K., Waterfield, T., Yelekçi, O., Yu, R., and Zhou, B., Cambridge University Press, Cambridge, United Kingdom and New York, NY, USA, 553–672, 2021.
Leggett, J., Pepper, W. J., Swart, R. J., Edmonds, J., Meira Filho, L., Mintzer, I., and Wang, M.: Emissions scenarios for the IPCC: an update, Climate change, 1040, 75–95, 1992.
Lehner, F., Deser, C., Maher, N., Marotzke, J., Fischer, E. M., Brunner, L., Knutti, R., and Hawkins, E.: Partitioning climate projection uncertainty with multiple large ensembles and CMIP5/6, Earth Syst. Dynam., 11, 491–508, https://doi.org/10.5194/esd-11-491-2020, 2020.
Liang, Y., Gillett, N. P., and Monahan, A. H.: Climate Model Projections of 21st Century Global Warming Constrained Using the Observed Warming Trend, Geophys. Res. Lett., 47, e2019GL086757, https://doi.org/10.1029/2019GL086757, 2020.
Liu, L., Shawki, D., Voulgarakis, A., Kasoar, M., Samset, B. H., Myhre, G., Forster, P. M., Hodnebrog, Ø., Sillmann, J., Aalbergsjø, S. G., Boucher, O., Faluvegi, G., Iversen, T., Kirkevåg, A., Lamarque, J.-F., Olivié, D., Richardson, T., Shindell, D., and Takemura, T.: A PDRMIP Multimodel Study on the Impacts of Regional Aerosol Forcings on Global and Regional Precipitation, J. Climate, 31, 4429–4447, https://doi.org/10.1175/JCLI-D-17-0439.1, 2018.
Liu, Q., Peng, C., Schneider, R., Cyr, D., McDowell, N. G., and Kneeshaw, D.: Drought-induced increase in tree mortality and corresponding decrease in the carbon sink capacity of Canada's boreal forests from 1970 to 2020, Glob. Change Biol., 29, 2274–2285, https://doi.org/10.1111/gcb.16599, 2023.
Lyon, C., Saupe, E. E., Smith, C. J., Hill, D. J., Beckerman, A. P., Stringer, L. C., Marchant, R., McKay, J., Burke, A., and O'Higgins, P.: Climate change research and action must look beyond 2100, Glob. Change Biol., 28, 349–361, 2022.
MacDougall, A. H., Frölicher, T. L., Jones, C. D., Rogelj, J., Matthews, H. D., Zickfeld, K., Arora, V. K., Barrett, N. J., Brovkin, V., Burger, F. A., Eby, M., Eliseev, A. V., Hajima, T., Holden, P. B., Jeltsch-Thömmes, A., Koven, C., Mengis, N., Menviel, L., Michou, M., Mokhov, I. I., Oka, A., Schwinger, J., Séférian, R., Shaffer, G., Sokolov, A., Tachiiri, K., Tjiputra , J., Wiltshire, A., and Ziehn, T.: Is there warming in the pipeline? A multi-model analysis of the Zero Emissions Commitment from CO2, Biogeosciences, 17, 2987–3016, https://doi.org/10.5194/bg-17-2987-2020, 2020.
Mace, M. J.: Mitigation Commitments Under the Paris Agreement and the Way Forward, Climate Law, 6, 21–39, https://doi.org/10.1163/18786561-00601002, 2016.
McKenna, C. M., Maycock, A. C., Forster, P. M., Smith, C. J., and Tokarska, K. B.: Stringent mitigation substantially reduces risk of unprecedented near-term warming rates, Nat. Clim. Change, 11, 126–131, https://doi.org/10.1038/s41558-020-00957-9, 2021.
Meinshausen, M., Smith, S. J., Calvin, K., Daniel, J. S., Kainuma, M. L., Lamarque, J.-F., Matsumoto, K., Montzka, S. A., Raper, S. C., and Riahi, K.: The RCP greenhouse gas concentrations and their extensions from 1765 to 2300, Climatic Change, 109, 213–241, 2011.
Meinshausen, M., Nicholls, Z. R. J., Lewis, J., Gidden, M. J., Vogel, E., Freund, M., Beyerle, U., Gessner, C., Nauels, A., Bauer, N., Canadell, J. G., Daniel, J. S., John, A., Krummel, P. B., Luderer, G., Meinshausen, N., Montzka, S. A., Rayner, P. J., Reimann, S., Smith, S. J., van den Berg, M., Velders, G. J. M., Vollmer, M. K., and Wang, R. H. J.: The shared socio-economic pathway (SSP) greenhouse gas concentrations and their extensions to 2500, Geosci. Model Dev., 13, 3571–3605, https://doi.org/10.5194/gmd-13-3571-2020, 2020.
Meinshausen, M., Lewis, J., McGlade, C., Gütschow, J., Nicholls, Z., Burdon, R., Cozzi, L., and Hackmann, B.: Realization of Paris Agreement pledges may limit warming just below 2 °C, Nature, 604, 304–309, 2022.
Melnikova, I., Boucher, O., Cadule, P., Ciais, P., Gasser, T., Quilcaille, Y., Shiogama, H., Tachiiri, K., Yokohata, T., and Tanaka, K.: Carbon Cycle Response to Temperature Overshoot Beyond 2 °C: An Analysis of CMIP6 Models, Earth's Future, 9, e2020EF001967, https://doi.org/10.1029/2020EF001967, 2021.
Mengel, M., Nauels, A., Rogelj, J., and Schleussner, C.-F.: Committed sea-level rise under the Paris Agreement and the legacy of delayed mitigation action, Nat. Commun., 9, 601, https://doi.org/10.1038/s41467-018-02985-8, 2018.
Moss, R. H., Edmonds, J. A., Hibbard, K. A., Manning, M. R., Rose, S. K., Van Vuuren, D. P., Carter, T. R., Emori, S., Kainuma, M., and Kram, T.: The next generation of scenarios for climate change research and assessment, Nature, 463, 747–756, 2010.
Nakicenovic, N., Alcamo, J., Davis, G., Vries, B. d., Fenhann, J., Gaffin, S., Gregory, K., Grubler, A., Jung, T. Y., and Kram, T.: IPCC Special report on emissions scenarios, edited by: Intergovernmental Panel on Climate Change, Intergovernmental Panel on Climate Change, Geneva, Switzerland, ISBN: 0 521 80081 1, 2000.
O'Neill, B. C., Tebaldi, C., van Vuuren, D. P., Eyring, V., Friedlingstein, P., Hurtt, G., Knutti, R., Kriegler, E., Lamarque, J.-F., Lowe, J., Meehl, G. A., Moss, R., Riahi, K., and Sanderson, B. M.: The Scenario Model Intercomparison Project (ScenarioMIP) for CMIP6, Geosci. Model Dev., 9, 3461–3482, https://doi.org/10.5194/gmd-9-3461-2016, 2016.
O'Neill, B. C., Kriegler, E., Riahi, K., Ebi, K. L., Hallegatte, S., Carter, T. R., Mathur, R., and Van Vuuren, D. P.: A new scenario framework for climate change research: the concept of shared socioeconomic pathways, Climatic Change, 122, 387–400, 2014.
O'Neill, B. C., Carter, T. R., Ebi, K., Harrison, P. A., Kemp-Benedict, E., Kok, K., Kriegler, E., Preston, B. L., Riahi, K., Sillmann, J., van Ruijven, B. J., van Vuuren, D., Carlisle, D., Conde, C., Fuglestvedt, J., Green, C., Hasegawa, T., Leininger, J., Monteith, S., and Pichs-Madruga, R.: Achievements and needs for the climate change scenario framework, Nat. Clim. Change, 10, 1074–1084, https://doi.org/10.1038/s41558-020-00952-0, 2020.
Otto, F. E., Minnerop, P., Raju, E., Harrington, L. J., Stuart-Smith, R. F., Boyd, E., James, R., Jones, R., and Lauta, K. C.: Causality and the fate of climate litigation: The role of the social superstructure narrative, Global Policy, 13, 736–750, 2022.
Palazzo Corner, S., Siegert, M., Ceppi, P., Fox-Kemper, B., Frölicher, T. L., Gallego-Sala, A., Haigh, J., Hegerl, G. C., Jones, C. D., Knutti, R., Koven, C. D., MacDougall, A. H., Meinshausen, M., Nicholls, Z., Sallée, J. B., Sanderson, B. M., Séférian, R., Turetsky, M., Williams, R. G., Zaehle, S., and Rogelj, J.: The Zero Emissions Commitment and climate stabilization, Front. Sci., 1, 1–26, https://doi.org/10.3389/fsci.2023.1170744, 2023.
Persad, G., Samset, B., Wilcox, L., Allen, R. J., Bollasina, M. A., Booth, B. B., Bonfils, C., Crocker, T., Joshi, M., and Lund, M. T.: Rapidly evolving aerosol emissions are a dangerous omission from near-term climate risk assessments, Environ. Res. Clim., 2, 032001, https://doi.org/1088/2752-5295/acd6af, 2023.
Persad, G. G., Samset, B. H., and Wilcox, L. J.: Aerosols must be included in climate risk assessments, Nature, 611, 662–664, 2022.
Pfleiderer, P., Schleussner, C.-F., Mengel, M., and Rogelj, J.: Global mean temperature indicators linked to warming levels avoiding climate risks, Environ. Res. Lett., 13, 064015, https://doi.org/10.1088/1748-9326/aac319, 2018.
Pfleiderer, P., Schleussner, C.-F., and Sillmann, J.: No going back – Limited reversibility of regional climate changes under overshoot, Authorea [preprint], https://doi.org/10.22541/au.168245326.65777676/v1, 2023.
Pirani, A., Fuglestvedt, J. S., Byers, E., O'Neill, B., Riahi, K., Lee, J.-Y., Marotzke, J., Rose, S. K., Schaeffer, R., and Tebaldi, C.: Scenarios in IPCC assessments: lessons from AR6 and opportunities for AR7, npj Climate Action, 3, 1, https://doi.org/10.1038/s44168-023-00082-1, 2024.
Rajamani, L., Jeffery, L., Höhne, N., Hans, F., Glass, A., Ganti, G., and Geiges, A.: National “fair shares” in reducing greenhouse gas emissions within the principled framework of international environmental law, Climate Policy, 21, 983–1004, https://doi.org/10.1080/14693062.2021.1970504, 2021.
Riahi, K., Van Vuuren, D. P., Kriegler, E., Edmonds, J., O'neill, B. C., Fujimori, S., Bauer, N., Calvin, K., Dellink, R., and Fricko, O.: The Shared Socioeconomic Pathways and their energy, land use, and greenhouse gas emissions implications: An overview, Global Environ. Chang., 42, 153–168, 2017.
Riahi, K., Schaeffer, R., Arango, J., Calvin, K., Guivarch, C., Hasegawa, T., Jiang, K., Kriegler, E., Matthews, R., Peters, G. P., Rao, A., Robertson, S., Sebbit, A. M., Steinberger, J., Tavoni, M., and van Vuuren, D. P.: Mitigation pathways compatible with long-term goals, in: Climate Change 2022: Mitigation of Climate Change, Contribution of Working Group III to the Sixth Assessment Report of the Intergovernmental Panel on Climate Change, edited by: Intergovernmental Panel on Climate Change, Cambridge University Press, Cambridge, UK and New York, USA, https://doi.org/10.1017/9781009157926.005, 2022.
Ribes, A., Qasmi, S., and Gillett, N. P.: Making climate projections conditional on historical observations, Sci. Adv., 7, eabc0671, https://doi.org/10.1126/sciadv.abc0671, 2021.
Richters, O., Bertram, C., Kriegler, E., Anz, J., Beck, T., Bresch, D., Charles, M., Clarke, L., Cui, R., Edmonds, J., Hackstock, P., Hilaire, J., Holland, D., Hurst, I., Khourdajie, A., Kikstra, J., Kropf, C., Lejeune, Q., Lewis, J., Liadze, I., Meinshausen, M., Min, J., Nicholls, Z., Pfleiderer, P., Piontek, F., Rogelj, J., Sauer, I., Schwind, N., Schleussner, C., Schleypen, J., Sferra, F., van Ruijven, B., Weigmann, P., Yu, S., Zhao, A., Zimmer, A., and Zwerling, M.: NGFS Climate Scenario Database: Technical Documentation V3.1, https://www.ngfs.net/sites/default/files/media/2022/11/21/technical _documentation_ngfs_scenarios_phase_3.pdf (last access: 3 May 2024), 2022.
Rogelj, J., Popp, A., Calvin, K. V., Luderer, G., Emmerling, J., Gernaat, D., Fujimori, S., Strefler, J., Hasegawa, T., Marangoni, G., Krey, V., Kriegler, E., Riahi, K., van Vuuren, D. P., Doelman, J., Drouet, L., Edmonds, J., Fricko, O., Harmsen, M., Havlík, P., Humpenöder, F., Stehfest, E., and Tavoni, M.: Scenarios towards limiting global mean temperature increase below 1.5 °C, Nat. Clim. Change, 8, 325–332, https://doi.org/10.1038/s41558-018-0091-3, 2018.
Rogelj, J., Fransen, T., den Elzen, M. G. J., Lamboll, R. D., Schumer, C., Kuramochi, T., Hans, F., Mooldijk, S., and Portugal-Pereira, J.: Credibility gap in net-zero climate targets leaves world at high risk, Science, 380, 1014–1016, https://doi.org/10.1126/science.adg6248, 2023.
Samset, B. H., Fuglestvedt, J. S., and Lund, M. T.: Delayed emergence of a global temperature response after emission mitigation, Nat. Commun., 11, 3261, https://doi.org/10.1038/s41467-020-17001-1, 2020.
Sanderson, B. M., Booth, B. B. B., Dunne, J., Eyring, V., Fisher, R. A., Friedlingstein, P., Gidden, M. J., Hajima, T., Jones, C. D., Jones, C., King, A., Koven, C. D., Lawrence, D. M., Lowe, J., Mengis, N., Peters, G. P., Rogelj, J., Smith, C., Snyder, A. C., Simpson, I. R., Swann, A. L. S., Tebaldi, C., Ilyina, T., Schleussner, C.-F., Seferian, R., Samset, B. H., van Vuuren, D., and Zaehle, S.: The need for carbon emissions-driven climate projections in CMIP7, EGUsphere [preprint], https://doi.org/10.5194/egusphere-2023-2127, 2023.
Santana-Falcón, Y., Yamamoto, A., Lenton, A., Jones, C. D., Burger, F. A., John, J. G., Tjiputra, J., Schwinger, J., Kawamiya, M., Frölicher, T. L., Ziehn, T., and Séférian, R.: Irreversible loss in marine ecosystem habitability after a temperature overshoot, Commun. Earth Environ., 4, 343, https://doi.org/10.1038/s43247-023-01002-1, 2023.
Schleussner, C.-F., Pfleiderer, P., Andrijevic, M., Vogel, M. M., Otto, F. E. L., and Seneviratne, S. I.: Pathways of climate resilience over the 21st century, Environ. Res. Lett., 16, 054058, https://doi.org/10.1088/1748-9326/abed79, 2021.
Schleussner, C.-F., Ganti, G., Rogelj, J., and Gidden, M. J.: An emission pathway classification reflecting the Paris Agreement climate objectives, Commun. Earth Environ., 3, 135, https://doi.org/10.1038/s43247-022-00467-w, 2022.
Schwinger, J. and Tjiputra, J.: Ocean carbon cycle feedbacks under negative emissions, Geophys. Res. Lett., 45, 5062–5070, 2018.
Seneviratne, S. I., Rogelj, J., Séférian, R., Wartenburger, R., Allen, M. R., Cain, M., Millar, R. J., Ebi, K. L., Ellis, N., Hoegh-Guldberg, O., Payne, A. J., Schleussner, C.-F., Tschakert, P., and Warren, R. F.: The many possible climates from the Paris Agreement's aim of 1.5 °C warming, Nature, 558, 41–49, https://doi.org/10.1038/s41586-018-0181-4, 2018a.
Seneviratne, S. I., Wartenburger, R., Guillod, B. P., Hirsch, A. L., Vogel, M. M., Brovkin, V., van Vuuren, D. P., Schaller, N., Boysen, L., Calvin, K. V., Doelman, J., Greve, P., Havlik, P., Humpenöder, F., Krisztin, T., Mitchell, D., Popp, A., Riahi, K., Rogelj, J., Schleussner, C.-F., Sillmann, J., and Stehfest, E.: Climate extremes, land-climate feedbacks and land-use forcing at 1.5 °C, Philos. T. A-Math. Phys. Eng. Sci., 376, 20160450, https://doi.org/10.1098/rsta.2016.0450, 2018b.
Seneviratne, S. I., Zhang, X., Adnan, M., Badi, W., Dereczynski, C., Di Luca, A., Ghosh, S., Iskandar, I., Kossin, J., Lewis, S., Otto, F., Pinto, I., Satoh, M., Vicente-Serrano, S. M., Wehner, M., and Zhou, B.: Weather and Climate Extreme Events in a Changing Climate, in: Climate Change 2021: The Physical Science Basis, Contribution of Working Group I to the Sixth Assessment Report of the Intergovernmental Panel on Climate Change, edited by: IPCC, Cambridge University Press, Cambridge, United Kingdom and New York, NY, USA, 1513–1766, https://doi.org/10.1017/9781009157896.013, 2021.
Shiogama, H., Watanabe, M., Yoshimori, M., Yokohata, T., Ogura, T., Annan, J. D., Hargreaves, J. C., Abe, M., Kamae, Y., O'ishi, R., Nobui, R., Emori, S., Nozawa, T., Abe-Ouchi, A., and Kimoto, M.: Perturbed physics ensemble using the MIROC5 coupled atmosphere–ocean GCM without flux corrections: experimental design and results, Clim. Dynam., 39, 3041–3056, https://doi.org/10.1007/s00382-012-1441-x, 2012.
Shiogama, H., Tatebe, H., Hayashi, M., Abe, M., Arai, M., Koyama, H., Imada, Y., Kosaka, Y., Ogura, T., and Watanabe, M.: MIROC6 Large Ensemble (MIROC6-LE): experimental design and initial analyses, Earth Syst. Dynam., 14, 1107–1124, https://doi.org/10.5194/esd-14-1107-2023, 2023.
Sigmond, M., Fyfe, J. C., Saenko, O. A., and Swart, N. C.: Ongoing AMOC and related sea-level and temperature changes after achieving the Paris targets, Nat. Clim. Change, 10, 672–677, https://doi.org/10.1038/s41558-020-0786-0, 2020.
Sillmann, J., Stjern, C. W., Myhre, G., Samset, B. H., Hodnebrog, Ø., Andrews, T., Boucher, O., Faluvegi, G., Forster, P., Kasoar, M. R., Kharin, V. V., Kirkevåg, A., Lamarque, J.-F., Olivié, D. J. L., Richardson, T. B., Shindell, D., Takemura, T., Voulgarakis, A., and Zwiers, F. W.: Extreme wet and dry conditions affected differently by greenhouse gases and aerosols, npj Clim. Atmos. Sci., 2, 24, https://doi.org/10.1038/s41612-019-0079-3, 2019.
Sippel, S., Meinshausen, N., Fischer, E. M., Székely, E., and Knutti, R.: Climate change now detectable from any single day of weather at global scale, Nat. Clim. Change, 10, 35–41, 2020.
Smith, D. M., Gillett, N. P., Simpson, I. R., Athanasiadis, P. J., Baehr, J., Bethke, I., Bilge, T. A., Bonnet, R., Boucher, O., and Findell, K. L.: Attribution of multi-annual to decadal changes in the climate system: The Large Ensemble Single Forcing Model Intercomparison Project (LESFMIP), Front. Climate, 4, 955414, https://doi.org/10.3389/fclim.2022.955414, 2022.
Supran, G., Rahmstorf, S., and Oreskes, N.: Assessing ExxonMobil's global warming projections, Science, 379, eabk0063, https://doi.org/10.1126/science.abk0063, 2023.
Szopa, S., Naik, V., Adhikary, B., Artaxo, P., Berntsen, T., Collins, W. D., Fuzzi, S., Gallardo, L., Kiendler-Scharr, A., Klimont, Z., Liao, H., Unger, N., and Zanis, P.: Short-Lived Climate Forcers, in: Climate Change 2021: The Physical Science Basis, Contribution of Working Group I to the Sixth Assessment Report of the Intergovernmental Panel on Climate Change, edited by: Masson-Delmotte, V., Zhai, P., Pirani, A., Connors, S. L., Péan, C., Berger, S., Caud, N., Chen, Y., Goldfarb, L., Gomis, M. I., Huang, M., Leitzell, K., Lonnoy, E., Matthews, J. B. R., Maycock, T. K., Waterfield, T., Yelekçi, O., Yu, R., and Zhou, B., Cambridge University Press, Cambridge, United Kingdom and New York, NY, USA, 817–922, https://doi.org/10.1017/9781009157896.008, 2021.
Tang, T., Shindell, D., Samset, B. H., Boucher, O., Forster, P. M., Hodnebrog, Ø., Myhre, G., Sillmann, J., Voulgarakis, A., Andrews, T., Faluvegi, G., Fläschner, D., Iversen, T., Kasoar, M., Kharin, V., Kirkevåg, A., Lamarque, J.-F., Olivié, D., Richardson, T., Stjern, C. W., and Takemura, T.: Dynamical response of Mediterranean precipitation to greenhouse gases and aerosols, Atmos. Chem. Phys., 18, 8439–8452, https://doi.org/10.5194/acp-18-8439-2018, 2018.
Tebaldi, C., Debeire, K., Eyring, V., Fischer, E., Fyfe, J., Friedlingstein, P., Knutti, R., Lowe, J., O'Neill, B., Sanderson, B., van Vuuren, D., Riahi, K., Meinshausen, M., Nicholls, Z., Tokarska, K. B., Hurtt, G., Kriegler, E., Lamarque, J. F., Meehl, G., Moss, R., Bauer, S. E., Boucher, O., Brovkin, V., Byun, Y. H., Dix, M., Gualdi, S., Guo, H., John, J. G., Kharin, S., Kim, Y., Koshiro, T., Ma, L., Olivié, D., Panickal, S., Qiao, F., Rong, X., Rosenbloom, N., Schupfner, M., Séférian, R., Sellar, A., Semmler, T., Shi, X., Song, Z., Steger, C., Stouffer, R., Swart, N., Tachiiri, K., Tang, Q., Tatebe, H., Voldoire, A., Volodin, E., Wyser, K., Xin, X., Yang, S., Yu, Y., and Ziehn, T.: Climate model projections from the Scenario Model Intercomparison Project (ScenarioMIP) of CMIP6, Earth Syst. Dynam., 12, 253–293, https://doi.org/10.5194/esd-12-253-2021, 2021.
Tebaldi, C., Snyder, A., and Dorheim, K.: STITCHES: creating new scenarios of climate model output by stitching together pieces of existing simulations, Earth Syst. Dynam., 13, 1557–1609, https://doi.org/10.5194/esd-13-1557-2022, 2022.
Terhaar, J., Frölicher, T. L., Aschwanden, M. T., Friedlingstein, P., and Joos, F.: Adaptive emission reduction approach to reach any global warming target, Nat. Clim. Change, 12, 1136–1142, https://doi.org/10.1038/s41558-022-01537-9, 2022.
Tokarska, K. B., Stolpe, M. B., Sippel, S., Fischer, E. M., Smith, C. J., Lehner, F., and Knutti, R.: Past warming trend constrains future warming in CMIP6 models, Sci. Adv., 6, eaaz9549, https://doi.org/10.1126/sciadv.aaz9549, 2020.
Tromp, T. K., Shia, R.-L., Allen, M., Eiler, J. M., and Yung, Y. L.: Potential environmental impact of a hydrogen economy on the stratosphere, Science, 300, 1740–1742, 2003.
UNFCCC: Decision 1/CMA.3 Glasgow Climate Pact 2021, https://unfccc.int/sites/default/files/resource/cma2021_10_add1_adv.pdf (last access: 3 May 2024), 2021.
UNFCCC: Synthesis report for the technical assessment component of the first global stocktake, UNFCCC, Bonn, Germany, 32, https://unfccc.int/documents/461517 (last access: 3 May 2024), 2022a.
UNFCCC: Decision 21/CP.27 Second periodic review of the long-term global goal under the Convention and of overall progress towards achieving it FCCC/CP/2022/10/Add.2, https://unfccc.int/sites/default/files/resource/cp2022_10a02_adv.pdf (last access: 3 May 2024), 2022b.
UNFCCC: Decision –/CMA.5 – Sixth Assessment Report of the Intergovernmental Panel on Climate Change, https://unfccc.int/sites/default/files/resource/cma5_auv_3a_ipcc.pdf (last access: 3 May 2024), 2023a.
UNFCCC: Decision /CMA.5, Outcome of the first global stocktake, https://unfccc.int/sites/default/files/resource/cma5_auv_4_gst.pdf (last access: 3 May 2024), 2023b.
van der Wijst, K.-I., Bosello, F., Dasgupta, S., Drouet, L., Emmerling, J., Hof, A., Leimbach, M., Parrado, R., Piontek, F., Standardi, G., and van Vuuren, D.: New damage curves and multimodel analysis suggest lower optimal temperature, Nat. Clim. Change, 13, 434–441, https://doi.org/10.1038/s41558-023-01636-1, 2023.
van Diemen, R., Matthews, J. B. R., Möller, V., Fuglestvedt, J. S., Masson-Delmotte, V., Méndez, C., Reisinger, A., and Semenov, S.: Annex I: Glossary, in: Climate Change 2022: Mitigation of Climate Change. Contribution of Working Group III to the Sixth Assessment Report of the Intergovernmental Panel on Climate Change, edited by: Shukla, P. R., Skea, J., Slade, R., Al Khourdajie, A., van Diemen, R., McCollum, D., Pathak, M., Some, S., Vyas, P., Fradera, R., Belkacemi, M., Hasija, A., Lisboa, G., Luz, S., and Malley, J., Cambridge University Press, Cambridge, UK and New York, USA, https://doi.org/10.1017/9781009157926.020, 2022.
van Vuuren, D., Tebaldi, C., and O'Neill, B. C.: ScenarioMIP SSC, and workshop participants: Pathways to next generation scenarios for CMIP7: ScenarioMIP workshop report, Zenodo, https://doi.org/10.5281/zenodo.8186116, 2023.
van Vuuren, D. P., Kriegler, E., O'Neill, B. C., Ebi, K. L., Riahi, K., Carter, T. R., Edmonds, J., Hallegatte, S., Kram, T., Mathur, R., and Winkler, H.: A new scenario framework for Climate Change Research: scenario matrix architecture, Climatic Change, 122, 373–386, https://doi.org/10.1007/s10584-013-0906-1, 2014.
Velders, G. J., Andersen, S. O., Daniel, J. S., Fahey, D. W., and McFarland, M.: The importance of the Montreal Protocol in protecting climate, P. Natl. Acad. Sci. USA, 104, 4814–4819, 2007.
Velders, G. J. M. and Daniel, J. S.: Uncertainty analysis of projections of ozone-depleting substances: mixing ratios, EESC, ODPs, and GWPs, Atmos. Chem. Phys., 14, 2757–2776, https://doi.org/10.5194/acp-14-2757-2014, 2014.
Warszawski, L., Frieler, K., Huber, V., Piontek, F., Serdeczny, O., and Schewe, J.: The inter-sectoral impact model intercomparison project (ISI–MIP): project framework, P. Natl. Acad. Sci. USA, 111, 3228–3232, 2014.
WCRP: A WCRP vision for accessible, useful and reliable climate modeling systems: Report of the Future of Climate Modeling Workshop, WCRP, online, https://www.wcrp-climate.org/WCRP-publications/2023/Final_Report_WCRP_FCM_Workshop.pdf (last access: 3 May 2024), 2023.
Westerling, A. L., Hidalgo, H. G., Cayan, D. R., and Swetnam, T. W.: Warming and Earlier Spring Increase Western U.S. Forest Wildfire Activity, Science, 313, 940–943, https://doi.org/10.1126/science.1128834, 2006.
World Meteorological Organization: Scientific Assessment of Ozone Depletion: 2022, World Meteorological Organization, Geneva, 509, https://csl.noaa.gov/assessments/ozone/2022/ (last access: 3 May 2024), 2022.
Wunderling, N., Winkelmann, R., Rockström, J., Loriani, S., Armstrong McKay, D. I., Ritchie, P. D. L., Sakschewski, B., and Donges, J. F.: Global warming overshoots increase risks of climate tipping cascades in a network model, Nat. Clim. Change, 13, 75–82, https://doi.org/10.1038/s41558-022-01545-9, 2023.
- Abstract
- Introduction
- Policy context for framing pathways
- Science questions for framing climate pathways
- A perspective on the next generation of framing climate pathways for ESM simulations
- Discussion
- Conclusions
- Data availability
- Author contributions
- Competing interests
- Disclaimer
- Acknowledgements
- Financial support
- Review statement
- References
- Abstract
- Introduction
- Policy context for framing pathways
- Science questions for framing climate pathways
- A perspective on the next generation of framing climate pathways for ESM simulations
- Discussion
- Conclusions
- Data availability
- Author contributions
- Competing interests
- Disclaimer
- Acknowledgements
- Financial support
- Review statement
- References