the Creative Commons Attribution 4.0 License.
the Creative Commons Attribution 4.0 License.
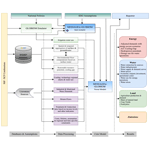
MESSAGEix-GLOBIOM nexus module: integrating water sector and climate impacts
Adriano Vinca
Edward Byers
Stefan Frank
Oliver Fricko
Esther Boere
Peter Burek
Miguel Poblete Cazenave
Paul Natsuo Kishimoto
Alessio Mastrucci
Yusuke Satoh
Amanda Palazzo
Madeleine McPherson
Keywan Riahi
Volker Krey
The integrated assessment model (IAM) MESSAGEix-GLOBIOM developed by IIASA is widely used to analyze global change and socioeconomic development scenarios within energy and land systems across different scales. However, to date, the representation of impacts from climate effects and water systems in the IAM has been limited. We present a new nexus module for MESSAGEix-GLOBIOM that improves the representation of climate impacts and enables the analysis of interactions between population, economic growth, energy, land, and water resources in a dynamic system. The module uses a spatially resolved representation of water systems to retain hydrological information without compromising computational feasibility. It maps simplified water availability and key infrastructure assumptions with the energy and land systems. The results of this study inform on the transformation pathways required under climate change impacts and mitigation scenarios. The pathways include multi-sectoral indicators highlighting the importance of water as a constraint in energy and land-use decisions and the implications of global responses to limited water availability from different sources, suggesting possible shifts in the energy and land sectors.
- Article
(5657 KB) - Full-text XML
-
Supplement
(1962 KB) - BibTeX
- EndNote
Multiple inter-sectoral objectives, including economic, environmental, and social goals, are integrated into formulating effective, sustainable policies over the long term. Nexus approaches have been increasingly used and considered in policy analysis, including the Sustainable Development Goals (SDGs), to exploit synergies and avoid negative trade-offs and unintended consequences considering the increased awareness of the interdependencies between the energy–water–land (EWL) sectors. Climate policy assessment helps identify pathways that can contribute toward achieving the “well below 2 °C” global warming target and other SDGs, such as food security and access to clean energy, water, and sanitation (Parkinson et al., 2018, 2019; Khan et al., 2017, 2018). In addition to climate change risks, limited resources compounded by population and GDP growth pose an additional challenge (Byers et al., 2018). Integrated assessment models (IAMs) help researchers and policymakers understand the long-term consequences of varying socioeconomic development and climate change scenarios. These scenarios assess the costs and benefits of climate change impacts and mitigation strategies. These models integrate sectors (global economy, energy, water, agriculture, and forestry) to provide policy insights relevant to climate change scenarios (Weyant, 2017). IAMs provide long-term transformation pathways to answer critical questions on climate change transition to ambitious climate policy goals (Riahi et al., 2017).
Substantial efforts have been made to develop scenarios that inform a range of future scenarios with varying societal and socioeconomic assumptions (Riahi et al., 2017). The most widely used set of scenarios in IAMs includes the Shared Socioeconomic Pathways (SSPs), a group of five quantified narratives for the evolution of socioeconomic development globally for the 21st century (O'Neill et al., 2017), and the Representation Concentration Pathways (RCPs), a set of four scenarios spanning a range of radiative forcing values (van Vuuren et al., 2011). These narratives have been translated into assumptions for economic growth, population change, and urbanization to analyze baseline and climate mitigation scenarios (Riahi et al., 2017).
Although SSPs were designed to analyze the challenges for mitigation and climate adaptation, the integration of climate impacts and the adaptation of energy and land sectors to water sector constraints have, until recently, been limited in the IAM scenarios due to substantial challenges in technical implementation and representation of climate impacts. Long-term assessment of climate mitigation scenarios often neglects the climate impacts on system performance, resulting in avoiding adaptation costs in the analysis (Calvin et al., 2013; Piontek et al., 2021). IAMs typically operate at a regional or continental scale to inform future pathways, whereas adaptation strategies require a more nuanced, localized focus emphasizing national and sub-national levels (Andrijevic et al., 2023). More detailed information on the spatial distribution of the costs and benefits of impacts and adaptation is required to inform adaptation actions and policies (Patt et al., 2010).
Impact modeling activities across diverse modeling groups, such as the Intercomparison Model Project (ISIMIP) (Frieler et al., 2017), have been carried out to better understand the impacts of climate change individually. These sectoral assessments evaluate biophysical impacts such as changing yields, runoff changes, food production, and groundwater. Economic impacts are subsequently estimated using various methodologies, chosen on the basis of the specific type of impact considered, such as the correlation between climate damages and temperature variations. Some studies have empirically linked climate conditions with socioeconomic systems and incorporated distributional factors into cost–benefit models, resulting in increased social costs of carbon and more stringent mitigation pathways (Parry and Carter, 2019; Howard and Sterner, 2017). Incorporating the representation of biophysical climate impacts into IAMs is crucial for understanding how various sectors influence techno-economic scenarios and for identifying appropriate mitigation and adaptation strategies (van Maanen et al., 2023; Andrijevic et al., 2023). Piontek et al. (2021) analyzed the economic impacts of climate change using the REMIND IAM model, but biophysical climate impacts were not represented. Soergel et al. (2021) emphasized the significance of considering the consequences of climate impacts and evaluating how integrated scenarios respond to these impacts, especially regarding sustainable development pathways. Schultes et al. (2021) highlighted the economic impact of climate change, advocating for immediate mitigation to reduce long-term damages and align with cost-effective Paris Agreement targets. This study proposes a framework incorporating high-resolution model outputs of biophysical climate impacts into IAMs, strengthening the water sector's resilience, and crafts scenarios with sustainable development objectives to evaluate climate change effects across various pathways, including mitigation, adaptation, and sustainability.
New analytical approaches and solutions are required to address the challenges of impacts and adaptation in long-term policy analysis (Wang et al., 2016; Patt et al., 2010; Riahi et al., 2017). There is a need for a balanced synthesis of Shared Socioeconomic Pathway (SSP) narratives with climate impacts, adaptation and resilience pathways to assess water, food, and energy security in order to assess sectoral adaptation costs and impacts (Rasul, 2016; Schleussner et al., 2021). Regions highly exposed to climate impacts, highly vulnerable populations (Byers et al., 2018), and developing regions face the biggest challenge in adapting to climate change impacts and simultaneously meeting growing population-driven demands in the EWL sectors (Rasul and Sharma, 2016). Integrating cross-sectoral EWL nexus analysis in IAMs can help identify trade-offs and synergies, integrate policy implementations, and address equity dimensions, such as the population exposed to hunger or lacking access to sanitation and electricity. This holistic approach is designed to elicit a model-endogenous response to climate impacts and SDGs constraints, thereby enhancing systemic resilience and advancing sustainable development. However, it does not delineate specific adaptation policies at the community level. Due to the spatial and temporal complexity of hydrological data, it is challenging to translate hydrological information into the IAMs. Usually, the spatial extent of IAMs is macro-regions, and the aggregated hydrological information loses adequate information at a macro-level. There is always a need to find a middle ground between showing the hydrological process more accurately and lowering the cost of computing (Fricko et al., 2016; Parkinson et al., 2019). There have been efforts to link a higher spatial resolution water sector to account for hydrological balance and constraints in IAMs, such as by Yates (1997) and Kim et al. (2016). Addressing the identified gaps, this study proposes a framework that integrates climate impacts with an emphasis on the water sector's role in climate change and develops scenarios in sync with sustainable development assumptions to evaluate the effects of climate change within the contexts of mitigation, adaptation, and sustainable development pathways.
This paper introduces a new module of the global MESSAGEix-GLOBIOM framework (Riahi et al., 2021; Krey et al., 2016). The nexus module attempts to fill the gap in integrated assessments by improving the representation of biophysical climate impacts across the EWL sectors and enhancing the water sector representation. We develop scenarios that can effectively capture climate impacts across multiple sectors using this module. Then, these scenarios are combined with SDG targets in EWL sectors to capture the synergies and trade-offs of climate impacts and sustainable development pathways.
The paper is structured as follows: Sect. 2 comprehensively explains the module's structure, improvements, and modular procedures. Details on specific components of the module, such as the water sector, biophysical climate impacts, SDGs, and flexibility at different scales (with Zambia as an example) are described in Sect. 3. Section 4 presents the results of the module's ability to answer different research questions, and Sect. 5 concludes with a summary of the study's significant findings and contributions.
Least-cost optimization using engineering-economic modeling is a common approach for long-term energy, water, and land planning (Barbier, 2012; Khan et al., 2017). However, it is not typically performed in a holistic manner that jointly considers system solutions across sectors in a single algorithm. These approaches have been a vital component of the MESSAGEix framework in analyzing a sustainable transition in climate change mitigation and sustainable socioeconomic development (Khan et al., 2018; Huppmann et al., 2019). Engineering-economic modeling methods to quantify the impacts, resource potential, and costs across different spatial and temporal scales are employed in the nexus module. The approach is both engineering and economical in scope because it combines physically based models of infrastructure systems with cost functions and decision rules for operation, expansion, and retirement at the process level through time. The theoretical underpinning of decision modeling is that system design choices are made at the least cost over the planning horizon in a perfectly foresighted, integrated way. The end-user prices for consumers are minimized, and flexibilities across sectors to absorb sectoral trade-offs are fully utilized and planned for in advance.
The “nexus” module of the MESSAGEix-GLOBIOM framework, MESSAGEix-GLOBIOM Nexus v1, presented in this paper contains endogenous spatially and temporally explicit climate impact constraints and water allocation algorithms. This module extends the foundational work carried out by Parkinson et al. (2019). It addresses the gaps in the previous study by improving the water sector resolution, water constraints, and climate impacts. The module here refers to expanding the core global framework of MESSAGEix-GLOBIOM to represent specific dimensions straightforwardly at the expense of increased computational complexity and cost. The MESSAGEix-GLOBIOM integrated assessment framework is a global energy–economic–agricultural–land-use model that evaluates the interconnected global energy systems, agriculture, land use, climate, and the economy. The MESSAGEix framework optimizes the total discounted system costs across all energy, land-use, and water sector representations using linear programming. It provides options for both perfect foresight and recursive dynamic modes. Its adaptability and flexibility make it a powerful instrument for optimizing transformation pathways at various scales, emphasizing the minimization of system costs. It comprises five complementary modules: the energy model MESSAGEix (Huppmann et al., 2019), the land-use model GLOBIOM (Havlík et al., 2014), the air pollution and greenhouse gas (GHG) model GAINS, the aggregated macro-economic model MACRO, and the simple climate model MAGICC (Meinshausen et al., 2011). The framework combines the MESSAGEix and GLOBIOM models to assess and model the economic, social, and environmental implications of policy scenarios. The framework comprehensively examines the trade-offs and synergies between numerous policy objectives, such as reducing greenhouse gas emissions, boosting food security, and safeguarding natural resources. To access sustainable development targets, the framework is utilized to evaluate the feasibility and implications of alternative policy choices and to guide decision-making.
The nexus module simultaneously determines the energy portfolio, land use, associated water requirements, and feedback from constrained resources, such as limited water availability for energy and land-use resource usage. It includes a framework for connecting information from hydrological models. It is designed to adapt any global hydrological model (GHM) output and be flexible across different spatial scales (regional definitions, global and country scales). A higher-resolution spatial layer at the basin scale is embedded within the module to retain valuable hydrological data. The information from the water sector is then mapped to the global MESSAGEix energy system at the MESSAGEix native region level. This connects valuable water resource data to the energy sectors and vice versa. The framework balances basin-level water availability and demand while mapping water necessary for energy and land usage at the MESSAGE native region level. The nexus module tracks annual municipal and industrial water demand, water required for power plant cooling technologies, energy extraction, and irrigation water use, balancing through water supply from several sources, such as surface water, groundwater, and desalinated water.
Furthermore, a wastewater treatment infrastructure representation tracks the water during collection, treatment, and reuse. Water demands are tracked across urban and rural components to enable a more comprehensive understanding of future development and adaptation needs. Additionally, biophysical climate impacts are integrated across EWL sectors, including water availability, desalination potential, hydropower potential, air-conditioning cooling demand, power plant cooling potential, and land-use variables (bioenergy, irrigation water) to account for the feedback associated with climate change within the module. GLOBIOM was also adjusted to capture water supply, availability, scarcity, and demand from other sectors based on GHM hydrological data under different climate-forcing scenarios. In this case, GLOBIOM and the MESSAGEix nexus module are configured to use outputs from gridded GHMs from the Inter-Sectoral Impact Model Intercomparison Project (ISIMIP) (Frieler et al., 2017). This information is specified for 210 river basins based on the HydroSHEDS basin delineation (Lehner et al., 2008) (Fig. 3).
One of the critical features of the nexus module is its ability to simulate global interactions across multiple sectors and systems. It allows the module to represent the complex feedback and spillover effects from policy interventions, such as the potential implications of land-use changes on the global food system and the energy sector or the water footprints of the energy system. The framework facilitates a comprehensive assessment of policy options by integrating scenario-based projections, including population and economic growth, technological advancements, and resource limitations.
The integrated approach thoroughly considers the trade-offs and synergies between diverse policy objectives, such as reducing greenhouse gas emissions, enhancing food security, and protecting natural resources. Considering biophysical climate impacts across different sectors helps to access different adaptation needs and responses in different sectoral outputs across different pathways. In the context of sustainable development, it can analyze the viability and implications of various policy alternatives and inform decision-making.
The MESSAGEix-GLOBIOM framework enables flexible integration with different modules, such as those on water, transport, materials, and buildings. The development process of the nexus module is divided into four phases: (i) identifying databases and literature studies for key assumptions; (ii) data processing to make the data model compatible; (iii) setting the core module, which compiles the data and populates it into the core model; and (iv) post-processing of the model outputs to provide ready-to-use results in a database and for visualization tools such as the IIASA scenario explorer (Huppmann et al., 2018).
The module uses SSP-RCP (Shared Socioeconomic Pathways – Representative Concentration Pathway) combinations as narratives for creating a baseline scenario. Each scenario is developed using SSP-RCP combinations, national policies, and Sustainable Development Goal (SDG) assumptions aggregated at the R11 region, a spatial delineation of 11 global regions used in the MESSAGEix-GLOBIOM. National policies, including energy use and emission trajectories, are formulated based on the existing MESSAGEix-GLOBIOM at 0.5°×0.5° spatial resolution, distributed monthly over the growing season based on local cropping calendars for a 10-year time step. These requirements are used as input to the GLOBIOM model. We used the global hydrological model (GHM) outputs from the ISIMIP database (Frieler et al., 2017) for water availability and hydropower potentials for biophysical impact indicators. The GLOBIOM model upscales these water requirements and provides irrigation requirements at an aggregated 37 regions based on land-use allocation decisions.
A typical scenario from the MESSAGEix-GLOBIOM is used to develop and extend the nexus module and consists of several crucial components (Riahi et al., 2021). Socioeconomic assumptions on population and GDP are used to form energy demand projections. Nationally determined contributions (NDCs) are applied to various sectors and configurations as policy implications, including but not limited to emission targets, energy shares, capacity or generation targets, and macro-economic targets. The reference energy system in this scenario features a comprehensive set of energy resources and conversion technologies from extraction to transmission and distribution. The outcome of this scenario estimates technology-specific multi-sector responses and pathways for various sectoral targets. The analysis is based on the Shared Socioeconomic Pathway (SSP) 2, which builds on historical trends as the starting point. The time horizon for the optimization framework of MESSAGEix-GLOBIOM extends from 2020 to 2100, with a non-regular distribution of time steps.
Further information on the typical scenarios of MESSAGEix-GLOBIOM can be found in Krey et al. (2016). The scenario is further extended from the typical scenario in the nexus module using certain policy and technological assumptions. The configuration can handle any SSP-RCP combinations to allow access to a diverse range of pathways compared to each other and the Reference scenario.
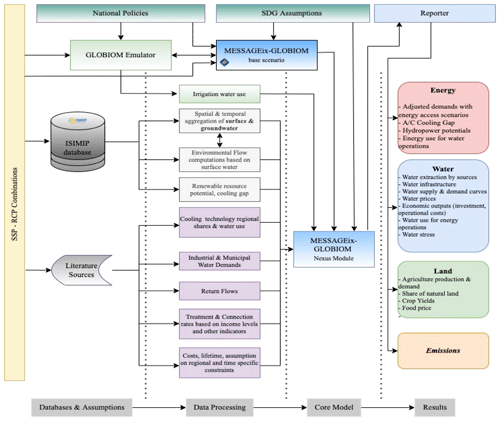
Figure 1Structure and data flows of the MESSAGEix-GLOBIOM nexus module. SSP-RCP combinations of scenarios are used as basis for the development of the nexus module. The module is built on the typical MESSAGEix-GLOBIOM scenario. The typical scenario has updated biophysical climate impacts in the energy and land sectors, and the water system is then added. The database assumptions, structure, and processing are the main components of this study besides the core model. Using the computational tools and post-processing methods, multidimensional sectoral results inform the pathways for different scenarios.
The subsequent sections explain the water resource structure (supply, demand, and infrastructure) of the modeling framework (Sect. 3.1), and Sect. 3.2 and 3.3 discuss the integration of biophysical climate impacts and SDG-related assumptions in the module.
3.1 Water resources and the water sector
The reference system for the water sector in the nexus module of MESSAGEix-GLOBIOM is shown in Fig. 3. This study applies the MESSAGEix-GLOBIOM (energy system model) in native R11 global macro-regions via its energy and land systems. The data sources used across the water sector are detailed in Table 1. The water sector loses important spatial information if aggregated on a macro scale. As a first step toward balancing water demand and supply, we have selected the HydroSHEDS river basin level 3 (Lehner et al., 2008), intersected with the R11 region and annual time step, as the ideal standard scale. This spatial layer results in 210 basins (B210, see Fig. 2), providing a more powerful depiction of the supply–demand system (Fig. 2). The energy demand for water uses and water withdrawals for irrigation and thermal power plant cooling are mapped from B210 to R11. This makes it possible to balance water supply and demand estimates at a suitable scale where the economic decision incorporates information on all processes, including water availability. We acknowledge that aggregating water needs across vast regions may underestimate the cascading effect of binding water limitations at the local level and the local-level adaptation components.
Using further high-resolution basin definitions adds additional complexity to the model due to upstream and downstream interdependence. Our initial 255 effort identifies the primary long-term regional and global drivers of gross imbalances in the supply and demand for water resources. Our ongoing research focuses on determining the most appropriate geographical (grid, sub-basin, or basin) and temporal (daily, monthly, or annual) scales for reconciling water demands and supplies in the global IAM for more robust climate extremes and adaptation needs. To better understand the spatial distribution and water balance of 260 regions, we can look at the Nile River basin, which extends across South Africa and the Middle East (R11 native regions). Due to the overlapping of these two R11 regions, we come up with two distinct spatial units: Nile–Middle East and Nile–South Africa. For Nile–South Africa, using proxy indicators such as the basin area and the proportion of available water in each basin, we now calculate the proportion of renewable water resources available from the Nile and the total water 265 availability in the South African region. This “downscaled” value plays a crucial role in the model, allowing us to reconcile the available water supply options with the region's varying water demands.
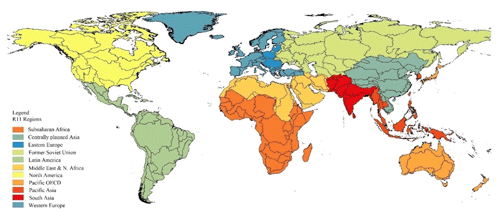
Figure 2Delineation of basins within the MESSAGE R11 regions. The HydroSHEDS basin level 3 is intersected with MESSAGE R11 regional delineation, and the new polygons are used as decision units in the water sector. The distinct colors in the map represent R11 regions; however, polygons inside each distinctly colored R11 region are the B210 basins intersected by the R11 region. The complete list of basin names along with the area in km2 can be found in the GitHub repository (https://github.com/iiasa/message-ix-models/blob/main/message_ix_models/data/node/B210-R11.yaml, last access: 24 March 2024).
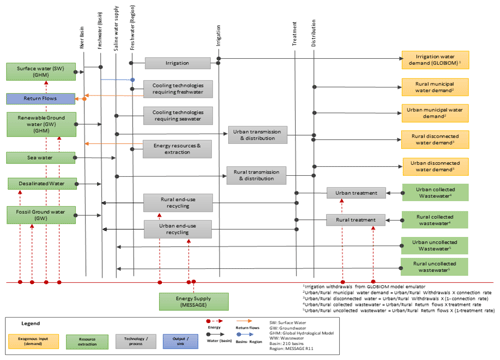
Figure 3Reference system of the water system representation in the nexus module. The arrows show the direction of the input/output of different technologies within the framework. The energy footprint of the water system is tracked at different supply steps and infrastructure technologies.
The water balance in the water sector of the IAM is
where Fr is the surface freshwater supplied from the river basin, Gw is freshwater supplied from groundwater aquifers, FGw is the non-renewable groundwater extractions, Ww is treated water provided from wastewater recycling facilities, D is desalinated water, Mc represents municipal and industrial sectoral demands, Irr defines the irrigation water withdrawals from the GLOBIOM emulator, and Ew is the water demand for the energy system. Irrigation and energy water demands are balanced at the regional level, and Ef is environmental flows calculated using the variable monthly flow (VMF) method (Fig. S3 in the Supplement) (Pastor et al., 2014). R represents MESSAGE energy regions. By contrast, B represents river basins within the given MESSAGE regions, and t represents time periods at a 5-year annual time interval. share is the share of freshwater in basins (B) per region (R) used as a proxy to balance irrigation and energy demands at the basin (B). All the values are in km3 yr−1. In GLOBIOM, irrigation water withdrawals are treated as residual claimants, with the water demands for the municipal and energy sectors taking priority (Palazzo et al., 2019; Frank et al., 2021). The water withdrawals are balanced with the supply of each model decision-making period and region.
Within the module, the choice between the supply system is motivated by the associated investments and operational costs. Renewable surface and groundwater freshwater are prioritized based on the cost. The other priority choice of supply between wastewater reuse, desalination, and fossil groundwater varies across regions, and the available potential in each region also varies. On the supply side, we use global gridded runoff and groundwater recharge data from the Community Water Model (CWatM) (Burek et al., 2020) and GHM outputs from ISIMIP (Frieler et al., 2017). Three bias-corrected meteorological forcing data from different climate models (GFDL-ESM2M, HadGEM2-ES, IPSL-CM5A-LR) are used to estimate surface runoff and groundwater recharge. We use multi-model ensemble mean runoff and groundwater recharge as an available renewable freshwater resource. We aggregate the gridded data (0.5°×0.5° spatial, daily time step) onto the B210 basins and 5-year annual average. For spatial aggregation, the spatial sum is used to sum the grid hydrological outputs (runoff and groundwater recharge) to the B210 basins. The detailed process is summarized in Table S2 in the Supplement.
We apply a quantile approach with monthly freshwater (surface and groundwater) resources for temporal aggregation to incorporate hydro-climate variability and prolonged dry periods. For example, for the 10th percentile, the monthly mean is first calculated from daily data. Then, we use the 10th percentile (Q90) of monthly freshwater runoff for a 20-year rolling window to determine a reliable flow for 90 % of the time. This type of percentile methodology applied to multi-decadal periods is frequently used in water resource and environmental flow assessments (Prudhomme et al., 2014; Satoh et al., 2022; Gleeson and Richter, 2018) to account for the seasonal low flows experienced in typical wet and average years, although not the driest 10 % of months (over 20 years). Figure S2 shows the Q90 flows overlaid on the monthly flow data for the significant basins to show their reliable flows. We have run the scenarios to test the model sensitivity based on the flow quantiles.
We followed the methodology described by Graham et al. (2020) to estimate the municipal water demands, where urban and rural components are derived from gridded population and income-level projections based on the SSPs, as detailed by Wang and Sun (2022). Manufacturing demands are generated following a similar approach used by Hejazi et al. (2014). Historical country-level data for 2015 are estimated by subtracting energy sector withdrawals from total industrial sector withdrawals. Future changes in manufacturing demands are projected, assuming convergence toward a log-linear model between GDP and manufacturing withdrawals. Demands are distributed across countries based on growth in GDP and then downscaled to 7.5 arcmin and re-aggregated at the B210 basins. Figures S5–S8 show the urban and rural components of municipal demands and industrial demands for 2050, while the data are provided in the GitHub repository (see Data Availability). Figures S5 and S6 show average municipal and industrial demands across the basins. The wastewater treatment system is adapted and improved from the previous implementation by Parkinson et al. (2019). Figure 3 shows the framework's conversion steps from wastewater collection to wastewater reuse. The module includes two generalized urban wastewater treatment technologies to simplify the number of decision variables. The first represents a standard secondary-level treatment facility commonly found in a mid-sized city.
By contrast, the second includes recycling capabilities and is parameterized to represent a standard facility suitable for upgrading municipal or manufacturing wastewater to potable standards, such as a membrane bioreactor. In addition, the module includes a rural wastewater treatment technology that meets the United Nations guidelines for clean water and sanitation in rural areas and is equivalent to a standard septic system. It ensures enough wastewater treatment capacity, including recycling and conventional treatment, to support the projected return flow connected to treatment. The desalination potentials have been estimated following the approach in Parkinson et al. (2019), where desalination capacity data are inferred against GDP trends using a logistic function. Here, data on water stress from Byers et al. (2018) have been added to the function to include the climate dimension in the projections (see Supplement Sect. S3.3).
We use the approach detailed by Fricko et al. (2016) to calculate water withdrawal and return flows from energy technologies. Each energy technology requiring water is provided with a withdrawal and consumption intensity (e.g., cubic kilometers per GWh). This allows the module to translate technology outputs into water requirements and return flows, which balance with the available supply. For power plant cooling technologies, where the water requirements are calculated as a function of heat rate, the efficiency change in the energy technologies (e.g., lower heat rates) impacts the cooling requirements per unit of electricity produced. The withdrawal and consumption intensities for power plant cooling technologies align with the range reported by Meldrum et al. (2013a). By contrast, the electricity balance computation includes additional electricity demands from recirculating and dry cooling technologies. Other technologies adhere to the data (Fricko et al., 2016)
The energy footprints of various components of the water sector, including supply (surface water and groundwater extraction), distribution (urban and rural), and wastewater treatment (treatment, recycling, and reuse), are interconnected with the electricity needs of the energy sector. This connection is established through basin-region mapping, which enables the spatial aggregation of appropriate fractions of electricity requirements to the region (R11) where the water sector's electricity consumption is managed. Table 1 indicates different references used for electricity requirements per unit of water infrastructure activity at different stages.
3.2 Climate impacts
The following climatic impacts are covered in the nexus module and this study: changes in crop yield, variations in precipitation patterns and drought severity, renewable energy potentials, cooling and heating energy demand, desalination potential, and cooling water discharge for energy use. Impacts on biodiversity are partially included in the evaluation whereby natural land serves as a high-level proxy indicator for the level of biodiversity. This method covers land-use change-induced consequences, which are the primary cause of biodiversity loss in the short term but it excludes direct climatic impacts. Thus, it primarily reflects the consequences of climate and SDG policies. All impact data are derived from the Intersectoral Model Intercomparison Project (ISIMIP) (Frieler et al., 2017) to maintain internal consistency across all indicators and models. The remainder of this section describes the model-specific representation of biophysical climate impacts across the energy, water, and land sectors and the methodological steps required to implement or update new climate impacts. We use the data for RCP2.6 and RCP6.0 to consider the climate impacts, i.e., emission pathways reaching 2.6 and 6.0 W m−2 forcing levels in 2100. We have not included GDP and labor productivity implications so as to focus solely on biophysical impacts.
The climate impacts on hydropower energy supply have been based on Gernaat et al. (2021). The difference between current and projected spatially explicit climate parameters is translated into spatially explicit energy supply estimates, which are translated into regional cost–supply curves. The climate data were used as input to calculate hydropower potential. It includes the theoretical potential of the upper limit of resource availability based on physical and hydrological conditions. The climate impacts were calculated for the historical and future periods using the ISIMIP database. The maps of technical potential, combined with economic information, have been used to generate cost–supply curves. These curves show the cumulative technical potential against the production cost, indicating that each location's production cost depends on its productivity. Cost–supply curves are widely used in IAMs to model the long-term cost development of renewable energy technologies. These curves indicate resource depletion, as the most productive sites are slowly being depleted, and thus higher-cost-incurring sites need to be used. On the other hand, note that climate impact on non-hydro renewables is not included in this study because excluding non-hydro renewables in the IAM is not expected to lead to significant discrepancies between the scenario results. Gernaat et al. (2021) have found relatively small impacts on renewable energy supply.
Regional cooling and heating demand days are based on the dataset and study by Byers et al. (2018), who derived their climate data from an ensemble of downscaled and bias-corrected global climate models (ISIMIP2). The data represent gridded global surface air temperature data at the daily resolution, summarized to decadal time steps and a monthly mean and subsequently aggregated to countries, weighted by SSP population. In this study, to estimate the corresponding energy demand in socioeconomic, technology, climate, and policy scenarios, we used two modules within the MESSAGEix-Buildings framework: CHILLED (Cooling and Heating gLobaL Energy Demand model), a bottom–up engineering model to estimate residential space heating and cooling energy demand; and STURM (Stock TURnover Model of Global Buildings), a stock turnover model based on dynamic material flow analysis (MFA) to assess the future evolution of the building stock (Mastrucci et al., 2021). The resulting estimates of the country's energy demand for cooling for SSP2 under RCP2.6 and RCP6.0 and the assumption of fixed historical temperature are aggregated from the country to the MESSAGE region. They are added to the module as a subcategory of the residential demand (Fig. S13).
Climate impacts on agriculture and the assessment of future hotspots are assessed in GLOBIOM by systematically integrating crop yield information from EPIC (Balkovič et al., 2014) (run for the different GCMs) for four crops (corn, wheat, maize, and rice) and applying it to our other crops using some assumption (Jägermeyr et al., 2021). The IIASA Global Forest Model (G4M) is used to model forest growth as a response to climate (Kindermann et al., 2008). The G4M uses a dynamic net primary productivity model to consider how growth rates are affected by changes in temperature, precipitation, radiation, and soil properties. G4M works with a monthly step, and the highest spatial resolution is 1 km2. The model estimates the impact on net primary productivity, mean annual increment, standing biomass, and harvestable biomass. Factor changes of mean annual increment and biomass accumulation under a certain degree of climate change compared to a scenario of no climate change are multiplied by the default rates in GLOBIOM. The GLOBIOM biophysical model incorporates agricultural yield, input requirements, and water availability for irrigation from the CWatM. This integration allows us to evaluate the relative effects of climate change on production, consumption, and market conditions and the autonomous adaptation to the impacts resulting from the GLOBIOM. Irrigation water withdrawals from the GLOBIOM are then linked to the nexus module, which balances the water system across other uncertainties.
3.3 SDGs
This section describes the energy, water, and land SDG measures in the module, which align with SDG2 (zero hunger, food access), SDG6 (clean water and sanitation, water access), SDG7 (affordable and clean energy, energy access), SDG15 (life on land, biodiversity). SDG13 (climate action) is also implicitly included in the framework when emission constraints are included in the scenario design. In this study, SDG13 is represented by achieving a 2.6 W m−2 (or a well below 2°) target in 2100. This is essentially the goal of the SDG – limiting climate change following the Paris Agreement. Table 3 provides an overview of all the (non-climate) nexus SDG measures, their representation in the modules, and the indicators to measure progress. The main criteria for including measures are as follows: (1) they should maximally benefit the overall goal, (2) they should be unambiguous and quantifiable, and (3) they should allow for consistent implementation across modules. The interaction between these measures and the other SDG categories is relatively limited.
The MESSAGE-Access-E-USE (end-use services of energy) model (Poblete-Cazenave and Pachauri, 2018; Poblete-Cazenave et al., 2021) is used for the analysis of household energy access to modern energy services for heating and cooking and has already been used on a global level to study demand in different socioeconomic pathways (Poblete-Cazenave and Pachauri, 2021; Pachauri et al., 2021). An estimation model takes as input micro-level data from nationally representative household surveys covering different regions of the world to estimate behavioral preference parameters that explain the choices of appliances and energy demands for different end uses based on household socioeconomic and demographic characteristics. Then, a simulation module uses the preference parameters estimated in the first module and additional external drivers that present potential pathways of socioeconomic growth and energy prices to simulate future appliance uptake and household energy demand under each scenario. This process is not internalized in MESSAGEix-GLOBIOM, but instead, a first iteration is performed to estimate the share of the population with access to modern energy sources for cooking (as opposed to traditional biomass or kerosene) given a fixed GDP pathway (SSP2) and energy prices related to each policy scenario. The model also assesses the implication of additional SDG policies regarding costs and transformations in the demand for energy. This is, however, separated from the solution of MESSAGE because an iterative procedure would alter the GDP pathways in the macro-economic component of the model (MACRO).
The SDG6 narrative is incorporated by applying supply and demand-side development across the water system. The supply-side measure includes constraints on available surface water as environmental flows. Maintaining environmental flows in rivers is instrumental in achieving SDG target 6.6, which aims to protect and restore water-related ecosystems, encompassing a range of natural landscapes from mountains and forests to wetlands, rivers, aquifers, and lakes. We use the variable monthly flow (VFM) method (Pastor et al., 2014) to constrain the monthly surface water available for human use based on environmental flow requirements (EFRs) for wet and dry seasons (Pastor et al., 2014). This method implies that water withdrawals cannot exceed the available residual supply after considering the EFRs. Some regions may be unable to adapt environmental flow targets in 2030 based on historical trajectories due to high withdrawals or fewer governance capabilities. We categorized these basins based on the development status of countries specified by the World Bank, implemented a lower environmental flow target in the respective regions from 2030 onward, and increased the target until 2050, thus following the trajectory of basins with high adaptive capacity. These environmental flow targets also vary across climate impact scenarios. This enables assessment of the response to mitigating future demand growth. The demand-side measures for SDG6 in the water system include targets for reaching sustainable water consumption across all sectors. We constrain the capacity of the water infrastructure system for integrating water access and quality targets. The connection and treatment rates are endogenized in the withdrawals and wastewater collection. These rates are changed to allow shifts in water withdrawals for universal piped access. Wastewater treatment capacity is increased to treat half of all the wastewater collection in the infrastructure system. The connection and treatment rates are adjusted for the basins that can readily adapt; the targets for 2030 are assigned to the basins with more adaptive capacity than those with less adaptive capacity. Increasing the fraction of wastewater treatment also helps to protect ecosystems related to water, thus contributing to achieving SDG target 6.6. The rates are projected in the baseline (non-SDG) scenario using a logistic model by combining income projections fitted to national historical data using the approach described in Parkinson et al. (2019).
The irrigation conservation approach is implemented to reduce the irrigation withdrawals and reallocate water to other sectors, thus contributing to target 6.4 (Frank et al., 2021). Pastor et al. (2019) mention how the reduced water approach in the irrigation sector in the GLOBIOM model accounts for environmental flows, and the water is reallocated to the environment and domestic uses by saving from the irrigation sector. The module chooses the irrigation water withdrawals based on the land-use emissions and associated costs to keep the land-related trade-offs with water and energy intact through the GLOBIOM emulator. The module enhancements do not cover all SDG6 targets, such as flood management and transboundary cooperation across basins. Concerning biodiversity protection, the GLOBIOM model assumes increased efforts and a doubling of the AICHI Biodiversity Target 11 (e.g., increase the total surface of protected areas to 17 % by 2030; Bacon et al., 2019). In addition, we use the UNEP-WCMC Carbon and Biodiversity Report (Kapos Ravilious et al., 2008) to identify highly biodiverse areas and prevent their conversion to agriculture or forest management from 2030 onward. We consider the area to be highly biodiverse where three or more biodiversity priority schemes overlap (Conservation International's Hotspots, WWF Global 200 terrestrial and freshwater ecoregions, Birdlife International Endemic Bird Areas, WWF/IUCN Centre of Plant Diversity and Amphibian Diversity Areas).
We estimate residential cooling gaps as the extent of the population needing space cooling without access and the additional energy demand required to close this gap and provide essential cooling comfort to all (Mastrucci et al., 2019). Minimum cooling requirements are calculated under the assumption of durable housing construction and conservative per-capita floor space and cooling operation to provide decent living standards (Kikstra et al., 2021), assuming the gap is covered with current cooling technologies, including fans and air conditioners.
Table 3SDG measures and indicators. Where possible and relevant, measures are fully implemented in 2030 and maintained until 2100 (see this https://sdgs.un.org/goals (last access: 24 March 2024) for SDG description).
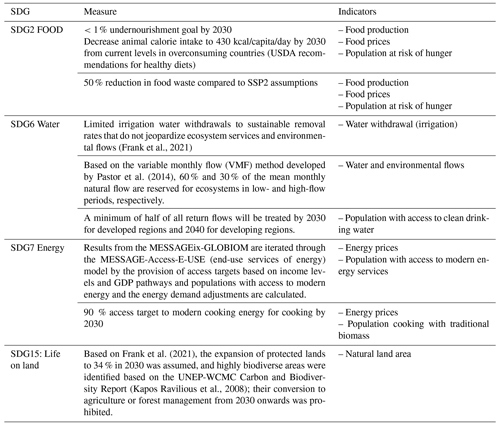
3.4 Flexibility across scales
As mentioned in Sect. 2, the module is flexible to adapt to a different spatial dimension with a higher resolution. In this case, we tested downscaling the global module for a particular country, Zambia. The energy sector is downscaled using the country model generator, which is used for various country-scale energy sector analyses, e.g., Orthofer et al. (2019). However, the nexus module also allows the water system to be prototyped rapidly for a country/basin level. The water reference system described in previous sections is pre-processed onto the higher-resolution spatial units from the gridded datasets and a base scenario is produced. The workflow diagram to produce the country-scale module is shown in Fig. S16. The Zambia-scale module is being used to develop an integrated platform combining different high-resolution sectoral models: the Water Crop Evapotranspiration model to estimate crop water demand for different crops (Tuninetti et al., 2015); an electricity demand assessment platform, M-LED, for communities without electricity supply (Falchetta et al., 2021); and the OnSSET tool to assess the least-cost electrification technologies and investment requirements based on electricity demand and energy potentials (Korkovelos et al., 2019). Falchetta et al. (2022) discuss the application of such linkages and provide further details.
In our current analysis, we applied the SSP2 framework in conjunction with both RCP2.6 and RCP6.0 to establish the current module setup. Future work will incorporate a broader array of SSPs paired with various RCPs to ensure a more comprehensive and coherent set of assumptions across different scenarios. Our examination of the biophysical effects of climate change on energy, water, and land-use sectors involved contrasting scenarios that integrate climate impacts – specifically designated as Impacts, Impacts-EN (focusing on the energy sector), Impacts-WAT (water sector), and Impacts-LU (land use) – alongside SDGs. We measured these against a reference scenario, which is predicated on historical climatic patterns and excludes any projections of climate impacts or SDG considerations. The scenario assumptions are detailed in Table 4.
Our study presents detailed results of water balance flows, providing a critical examination of global water management and the interdependencies within the water, energy, and land nexus. By comparing our module's outputs with benchmark values from the literature, we establish a validation baseline for EWL indicators, ensuring our findings resonate with recognized global estimates. Our study enables the monitoring of water balance flows at varying stages, offering an in-depth understanding of global water management and the intricate nexus between water, energy, and land. These interactions are depicted in Fig. 5a in the form of a Sankey diagram, along with input details and assumptions expounded in Sect. 3.1. The module provides a nuanced perspective, capturing the complexities of water resources and their utilization at both global and basin scales. Compared to the literature, global water resources (total runoff) are in the range of approximately 47 220 km3 yr−1, aligning with those reported by Burek et al. (2020) and Sutanudjaja et al. (2018). Across our module's scenarios, water withdrawals or water extractions fell within the 3365–3656 km3 yr−1 range, echoing figures found in established literature (Table 5). In our module, global wastewater collection is considered an exogenous input, quantified at approximately 310 km3 yr−1 for 2020, a figure that is broadly in line with the estimates from Jones et al. (2021). Global wastewater treatment volumes range from 156 to 172 km3 yr−1, in close agreement with the 187 km3 yr−1 reported by Jones et al. (2021). For agricultural water withdrawals, an essential water use sector, our module's estimate is 2670 km3 yr−1, which surpasses the 1250–2000 km3 yr−1 range reported by Burek et al. (2020), yet it is quite consistent with the 2735 km3 yr−1 figure suggested by Sutanudjaja et al. (2018). Figure 5b shows a range of water supply portfolios with varying water demands. Even though renewable energy sources are crucial overall, the makeup of these portfolios shows significant regional variation (Sects. S3 and S4 in the Supplement). Characterizing supply portfolios across various river basins will be the focus of future research projects under varying scenarios and water supply reliability levels. However, this structure allows us to see the water management portfolios linked with the energy and land sectors under varying climate and sustainable development scenarios.
Sectoral withdrawals primarily drive water extraction by source, with irrigation withdrawals from the GLOBIOM model making up a sizable portion. Figure S11 depicts the outlook for water extraction under the reference scenario. The effects of climate on crop yields show variability, with sugar crops experiencing a significant impact at 16 %, while cereals exhibit a comparatively modest change of approximately 1 %. The net yield effect is directly influenced by the intensity of nitrogen and phosphorus fertilization, which enhances water use efficiency and consequently reduces the demand for irrigation water. Furthermore, in our climate impact scenarios, increased CO2 levels also increase crop yields and contribute to improved water use efficiency, which is factored into our results. However, these results require cautious interpretation because our study did not account for cultivar optimization. The results affect water withdrawals and consequently influence the portfolio of water supplies. It is essential to highlight the role of enhanced irrigation efficiency assumptions in the SDG scenario, which results in a 29 % average reduction in total water withdrawals compared to climate impacts concurrent with the study by Frank et al. (2021). In addition, these effects contribute to a 28 % decrease in the marginal price of potable water due to adaptive responses to climate change impacts in electricity and irrigation withdrawals. By contrast, pursuing the SDGs can result in a significant price increase due to the increased allocation to environmental flows.
The results demonstrate that renewable surface water and groundwater are limited and vary across different climate scenarios. These effects decrease renewable water consumption, which is more evident in the land sector than in the water sector. In addition, our module indicates an increase in the use of alternative water sources such as brackish water, effluent, and desalination in certain regions, indicating that renewable water resources are limited in these areas. These observations highlight the significance of the SDGs further. For instance, when aligned with SDG6 targets, the module estimates a 24 % reduction in water consumption, resulting in a more sustainable water allocation to environmental flows (Fig. 4).
Figure 5 presents a comparative analysis of key energy–water–land (EWL) indicators across a spectrum of modeled scenarios. The boxplot distributions visually depict selected model output indicators for the period 2030–2080, covering scenarios such as Reference, Impacts, Impacts_LU (land use), Impacts_EN (energy), Impacts_WAT (water), and SDGs. The graph's constant trend in energy-related metrics across scenarios stands in stark contrast to the pronounced unpredictability of non-renewable water usage, suggesting that energy indicators are less vulnerable than water and land indicators.
Figure 5 also shows that, despite the biophysical impacts, agricultural production does not vary much. The SDG scenario, however, results in a considerable 20 % decrease in agricultural output, with the biophysical implications of land usage having a particular influence on sugar crop yields. This noteworthy effect emphasizes how susceptible some crops are to changes in land use and how crucial it is to take these effects into account when developing agricultural plans and policies.
Furthermore, the primary cause of the decrease in water withdrawals is the consequences of land use, wherein CO2 fertilization effects are a major factor. These effects on land usage decrease the overall need for irrigation and increase the efficiency with which agricultural operations use water.
Additionally, the figure also indicates that the cost of potable water has increased by 80 %, primarily due to the adoption of environmental flow allocations aimed at protecting freshwater ecosystems and the increased expenses linked to sophisticated wastewater treatment procedures. These elements highlight the intricate relationship that exists between water resource management and economic results as well as environmental care. The geophysical features and land-use influences of various regions mostly determine the global consequences of climate change on the water sector, with certain areas experiencing gains while others may have negative effects. Adaptive responses to climatic impacts reduce the number of people exposed to hunger by an average of 11 % according to the study. This is not as significant as the 30 % reduction in the SDG scenario, which is based on specific actions to reduce the risk of hunger.
It is imperative to exercise caution when interpreting the outcomes of the different scenarios, considering their reliance on several assumptions and their suitability for particular geographical and temporal circumstances. However, these results offer insightful information about the possible financial effects of various water management techniques. Different modeling methodologies may produce different results because assumptions, data inputs, and other elements are inherently variable. It is feasible to determine the most effective and successful tactics and to obtain a more thorough understanding of the probable consequences of different water management systems by comparing the outcomes from many models.
Table 5Comparison of EWL indicator results for the year 2020 with published literature sources for module validation.
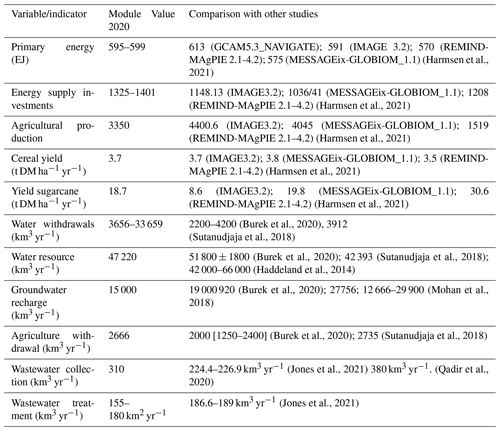
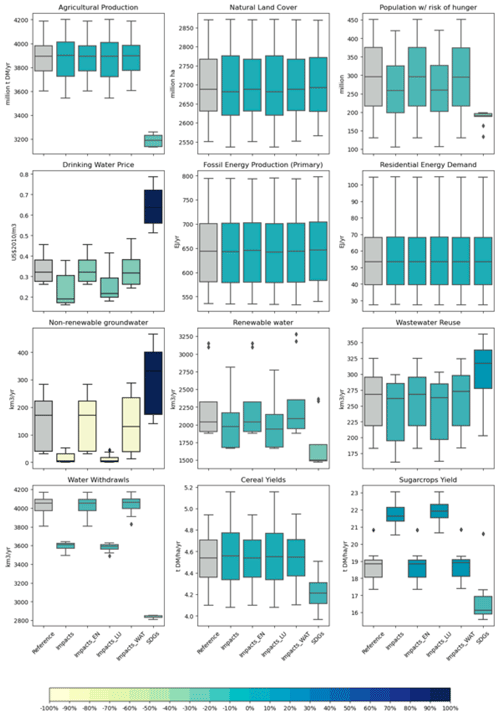
Figure 4A comparison of key EWL indicators across multiple scenarios showing the boxplot distributions for selected indicators from the module output. From 2030 to 2080, these are displayed against five distinct scenarios: reference, impacts, impacts_LU, impacts_EN, impacts_WAT, and SDGs. The reference scenario, which stands out visually with the gray hue, serves as a benchmark for other scenarios. The variance in color between the remaining boxplots represents the percentage change from the reference scenario.
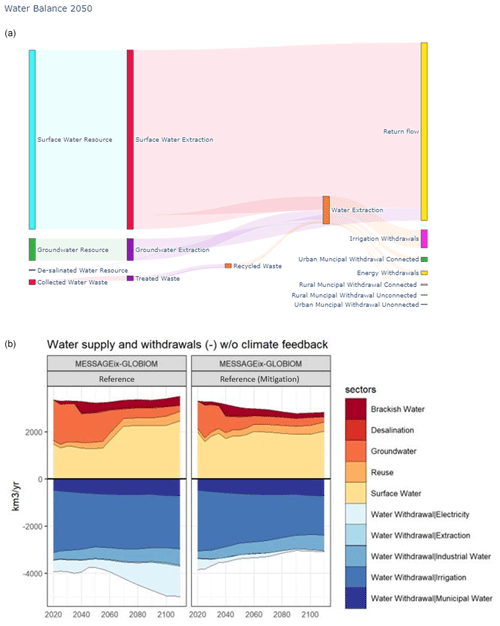
Figure 5(a) Water flows from supply to source in the water sector of the MESSAGEix-GLOBIOM nexus module. The flows and associated techno-economic parameters can be tracked as module outputs across the time horizon and scenarios. (b) The supply and withdrawal components of the global water balance, which are reported from the module outputs for the reference and impact scenarios. A range of blue hues are used to represent the supply sources, and a range of red hues are used to represent the withdrawals.
The MESSAGEix-GLOBIOM nexus module generates outputs that enhance our understanding of the complex interconnections of water, energy, and land, spanning from specific basins to the global scale. The outputs include assessments of water availability, indicators for Sustainable Development Goals (SDGs), and climate impacts unique to different sectors. These outputs serve as the foundation for conducting integrated route analysis. Figure 6 is a concise representation of the various outputs that can be generated by the module, emphasizing its ability to provide a wide range of scenario combinations. These combinations reveal the fundamental sensitivities and assumptions of various approaches, enabling us to identify effective methods that are adaptable to change and meet the needs of stakeholders.
To determine the effectiveness of the module in different climate and SDG scenarios, we developed a set of scenarios based on different assumptions. While theoretically impractical, the reference scenario acts as a benchmark for determining the outcomes of biophysical impacts by extrapolating previous climatic data into the future. The module also provides crucial investment and capacity projections at 5-year intervals, offering insights into the future of water management. In addition, we compared these indicators with the available literature in Table 5, confirming the dependability of our findings. This research provides a thorough understanding of global water, energy, and land interconnections. It has the potential to influence policy and investment choices, guiding us toward the sustainable use of resources.
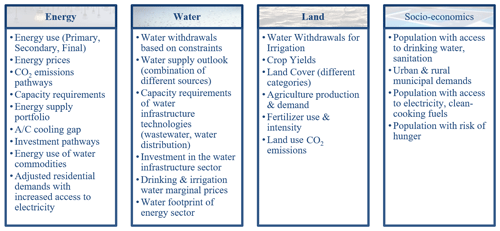
Figure 6Summary of output indicators that are possible from the MESSAGEix-GLOBIOM nexus module. These outputs are long-term pathways and many of these outputs can be further disaggregated on the technology level.
Our module effectively addresses the ever-changing climate system by utilizing a combination of internal and external outputs. As an example, we utilize EPIC to gain valuable information about how irrigation affects crop yields. These findings contribute to GLOBIOM, a system that adjusts land-use allocations based on the impacts of climate change. The reallocations, namely, in the utilization of land for irrigation, contribute to the balancing of water supplies in MESSAGEix-GLOBIOM. This balancing takes into consideration the requirements of different sectors in the face of changing climate conditions.
The reactions in the water sector are determined by the availability of resources. Climate-change-induced alterations in the water cycle determine how resources are distributed and require alternative sources to be found. The energy industry is subject to similar levels of dynamism, as climate changes have an impact on the efficiency of thermal power plants and the feasibility of hydropower projects while also increasing the demand for cooling. Our module provides a comprehensive multi-sectoral assessment by considering these biophysical consequences.
Understanding the interconnectedness of climate impacts across all sectors is essential; the ripple effects they cause require a comprehensive perspective. The results of our study emphasize the need for additional research to fully understand the range of potential effects that climate change could have on many industries and how their inclusion could greatly influence scenarios for managing and reducing these effects. The adoption of sustainable energy sources in certain areas demonstrates the wider significance of our research, which reveals the interaction between climate effects and strategies for reducing them, along with their additional benefits, such as improved agricultural output and a transition from fossil fuels in the power industry.
Our forthcoming research will expand on these preliminary findings, offering insights that are pertinent to policy-making. Upcoming articles will explore the integration of SDGs with climate policies, providing a fresh outlook on how to tackle climate adaptation problems effectively. While previous research has included SDG components in IAMs, our approach stands out by simultaneously analyzing SDG policies, climate targets, and impacts. This provides a new perspective on the climate adaptation narrative. We utilize this novel methodology to analyze the regional discrepancies in development objectives, facilitating our comprehension of how diverse regions might effectively manage the consequences of climate change while attaining their development targets. The study's regional insights will enhance our understanding of the adaptive strategies that regions may employ to achieve their developmental goals.
To summarize, the outputs of our module connected to the SDGs have the potential to greatly transform our understanding of human development indicators on a global and regional scale. Through the examination of metrics and the comparison of scenarios with and without SDGs, as illustrated in Fig. 7, we emphasize the novelty of integrating SDG scenarios with climate effect evaluations. This comprehensive scenario will support future studies, allowing us to assess the combined effects of actions to reduce and adapt to climate change in order to achieve sustainable development goals.
5.1 Further developments
While the module includes a detailed implementation of the water sector and a representation of biophysical climate impacts, we identify areas where our module lacks certain aspects and uncertainties. Since we look at the integrated systems, we do not include inter-basin or spatial unit transfers, which can be crucial for answering transboundary challenges in the river basins. Moreover, we currently do not account for water storage, a potentially important aspect of water resource management where we can see the water storage during a high-flow season and its use during a low-flow season. We use the flow percentiles approach to partially address this concern.
While the nexus module employs the robust outputs of the ISIMIP for depicting climate impacts, there are certain challenges from the current set of outputs that are not fully consistent with the input climate scenario assumptions. As soon as updated and aligned ISIMIP outputs become available, we will conduct a new model run to enhance consistency and reduce uncertainty in our analysis. In addition, the sensitivity of indicators to these impacts and the uncertainty of the global hydrological model (GHM) are more significant than those of climate models. The module's representation of alternative water constraints, such as the economic consequences of fossil groundwater extraction to reduce water consumption, will be explored in future research by focusing on more realistic groundwater assumptions. The current module structure, which assumes an endogenous adaptation response, may not fully capture the complex dynamics, such as the feedback mechanisms between water availability and energy production, the socioeconomic impacts of water scarcity on land use, and the long-term societal adaptations to water stress within the EWL sectors. Future research will focus on integrating these inter-sectoral feedback and dynamic responses to enhance the module's accuracy in depicting the intricacies of the EWL nexus.
In future research, we plan to expand our exploration of climate impact dimensions to include a more robust handling of statistical climate extremes, aiming for greater resilience in our module's performance at sub-annual temporal resolutions. Future versions of the module will integrate up-to-date climate impact data and strive for more consistent data sources across sectors.
In addition, we aim to distinguish the roles of impacts and adaptation responses within the EWL sectors, which will allow for a better understanding of the role of climate and the responses triggered by these impacts in the models. This future work will contribute to the module's refinement and expansion, resulting in a more comprehensive and accurate representation of the intricate interplay between climate impacts, water policy, and reliability.
This study addresses the research gap related to the improved EWL nexus, including biophysical climate impact representation within IAMs, by developing a nexus module for the global MESSAGEix-GLOBIOM IAM. It enhances the MESSAGEix framework to study the responses to biophysical climate impacts and water constraints across different scales. Representation of interactions with the water sector has been enhanced by implementing endogenous water sector spatial resolution and water constraints by balancing supply and demand at basin scales globally. It can address nexus synergies and trade-offs across EWL sectors on a global scale, showing regional results.
Moreover, the study shows that regional differences influence the cost of alternate water sources and infrastructure. Furthermore, the research on climate impacts highlights the biophysical consequences of climate change on many sectors and the necessity for additional research to comprehend their prospective outcomes. The study also investigates the effects of climate change on the power generation mix, highlighting the transition from fossil to renewable technologies. The results suggest that integrating biophysical repercussions can considerably impact the outcomes of climatic scenarios, and these findings should be regarded in the context of the entire model.
The module is improved to implement river ecosystem constraints, increasing socioeconomic demands, and ecological uncertainties. The module is developed consistent with state-of-the-art software development practices. The whole framework is transparent and flexible to be downscaled to any basin or country worldwide. A first-order module can be rapidly prototyped and further used to answer cutting-edge policy questions on the impacts and adaptation potentials across different basins, utilizing a set of socioeconomic and climate ensemble scenarios. The research will address the EWL nexus dynamics and interactions in terms of costs and structural changes concerning future resilient pathways.
The code, processed data, and documentation are available at https://doi.org/10.5281/zenodo.7687578 (Awais et al., 2023).
The supplement related to this article is available online at: https://doi.org/10.5194/gmd-17-2447-2024-supplement.
MA, AV, EB, VK, and KR conceived the modeling framework. MA and AV led the model development with support from EB, OF, PNK, KR, and VK. SF, EB, and AM helped with the GLOBIOM scenarios. AM provided data on cooling gaps, MPC ran energy access scenarios, and PB and YS provided the hydrological data. MM, KR, and VK did overall supervision. MA handled the manuscript and preparation of results. AV and EB coordinated the overall research. All authors reviewed and contributed to the manuscript writing.
The contact author has declared that none of the authors has any competing interests.
Publisher’s note: Copernicus Publications remains neutral with regard to jurisdictional claims made in the text, published maps, institutional affiliations, or any other geographical representation in this paper. While Copernicus Publications makes every effort to include appropriate place names, the final responsibility lies with the authors.
We thank Michelle van Vliet for providing the data for climate impacts on cooling technology and Michaela Werning for helping with spatial processing for the desalination data. We also acknowledge funding provided by the NAVIGATE project (H2020/2019-2023, grant agreement number 821124) and LEAP-RE (grant number 963530) of the European Commission. Yusuke Satoh was supported by Brain Pool Plus program funded by the Ministry of Science and ICT through the National Research Foundation of Korea(NRF-2021H1D3A2A03097768) and (NRF-2023R1A2C1004754).
This research has been supported by the Horizon 2020 (grant no. 821124), the NAVIGATE project (H2020/2019-2023, grant agreement number 821124), and LEAP-RE (grant number 963530) of the European Commission.
This paper was edited by Jinkyu Hong and reviewed by Page Kyle and one anonymous referee.
Andrijevic, M., Crespo Cuaresma, J., Muttarak, R., and Schleussner, C. F.: Governance in socioeconomic pathways and its role for future adaptive capacity, Nat. Sustain., 3, 35–41, https://doi.org/10.1038/s41893-019-0405-0, 2020.
Andrijevic, M., Schleussner, C. F., Crespo Cuaresma, J., Lissner, T., Muttarak, R., Riahi, K., Theokritoff, E., Thomas, A., van Maanen, N., and Byers, E.: Towards scenario representation of adaptive capacity for global climate change assessments, Nat. Clim. Change, 13, 778–787, https://doi.org/10.1038/s41558-023-01725-1, 2023.
Awais, M., Vinca, A., Byers, E., Frank, S., Fricko, O., Boere, E., Burek, P., Poblete Cazenave, M., Kishimoto, P. N., Mastrucci, A., Satoh, Y., Palazzo, A., McPherson, M., Riahi, K., and Krey, V.: MESSAGEix-GLOBIOM Nexus Module (v0.1), Zenodo [code, data set], https://doi.org/10.5281/zenodo.7687578, 2023.
Bacon, E., Gannon, P., Stephen, S., Seyoum-Edjigu, E., Schmidt, M., Lang, B., Sandwith, T., Xin, J., Arora, S., Adham, K. N., Espinoza, A. J. R., Qwathekana, M., Prates, A. P. L., Shestakov, A., Cooper, D., Ervin, J., Dias, B. F. de S., Leles, B., Attallah, M., Mulongoy, J., and Gidda, S. B.: Aichi Biodiversity Target 11 in the like-minded megadiverse countries, J. Nat. Conserv., 51, 125723, https://doi.org/10.1016/J.JNC.2019.125723, 2019.
Balkovič, J., van der Velde, M., Skalský, R., Xiong, W., Folberth, C., Khabarov, N., Smirnov, A., Mueller, N. D., and Obersteiner, M.: Global wheat production potentials and management flexibility under the representative concentration pathways, Global Planet. Change, 122, 107–121, https://doi.org/10.1016/j.gloplacha.2014.08.010, 2014.
Barbier, E. B.: The green economy post Rio+ 20, Science, 338, 887–888, 2012.
Burek, P., Greve, P., Wada, Y., Krey, V., Fischer, G., Parkinson, S., Tramberend, S., Byers, E., Satoh, Y., Riahi, K., Veldkamp, T. I. E., Burtscher, R., Djilali, N., Langan, S., and Kahil, T.: A Continental-Scale Hydroeconomic Model for Integrating Water-Energy-Land Nexus Solutions, Water Resour. Res., 54, 7511–7533, https://doi.org/10.1029/2017wr022478, 2018.
Burek, P., Satoh, Y., Kahil, T., Tang, T., Greve, P., Smilovic, M., Guillaumot, L., Zhao, F., and Wada, Y.: Development of the Community Water Model (CWatM v1.04) – a high-resolution hydrological model for global and regional assessment of integrated water resources management, Geosci. Model Dev., 13, 3267–3298, https://doi.org/10.5194/gmd-13-3267-2020, 2020.
Byers, E., Gidden, M., Leclere, D., Balkovic, J., Burek, P., Ebi, K., Greve, P., Grey, D., Havlik, P., Hillers, A., Johnson, N., Kahil, T., Krey, V., Langan, S., Nakicenovic, N., Novak, R., Obersteiner, M., Pachauri, S., Palazzo, A., Parkinson, S., Rao, N. D., Rogelj, J., Satoh, Y., Wada, Y., Willaarts, B., and Riahi, K.: Global exposure and vulnerability to multi-sector development and climate change hotspots, Environ. Res. Lett., 13, 055012, https://doi.org/10.1088/1748-9326/aabf45, 2018.
Calvin, K., Wise, M., Clarke, L., Edmonds, J., Kyle, P., Luckow, P., and Thomson, A.: Implications of simultaneously mitigating and adapting to climate change: initial experiments using GCAM, Clim. Change, 117, 545–560, 2013.
Dai, Z., Wu, Z., Loew, A., Jaramillo, P., and Zhai, H.: Marginal costs of water savings from cooling system retrofits: a case study for Texas power plants, Environ. Res. Lett, 11, 104004, https://doi.org/10.1088/1748-9326/11/10/104004, 2016.
Falchetta, G., Stevanato, N., Moner-Girona, M., Mazzoni, D., Colombo, E., and Hafner, M.: The M-LED platform: advancing electricity demand assessment for communities living in energy poverty, Environ. Res. Lett., 16, 074038, https://doi.org/10.1088/1748-9326/AC0CAB, 2021.
Falchetta, G., Adeleke, A., Awais, M., Byers, E., Copinschi, P., Duby, S., Hughes, A., Ireland, G., Riahi, K., Rukera-Tabaro, S., Semeria, F., Shendrikova, D., Stevanato, N., Troost, A., Tuninetti, M., Vinca, A., Zulu, A., and Hafner, M.: A renewable energy-centred research agenda for planning and financing Nexus development objectives in rural sub-Saharan Africa, Energy Strateg. Rev., 43, 100922, https://doi.org/10.1016/J.ESR.2022.100922, 2022.
Fan, Y., Li, H., and Miguez-Macho, G.: Global patterns of groundwater table depth, Science, 339, 940–943, https://doi.org/10.1126/science.1229881, 2013.
Frank, S., Gusti, M., Havlík, P., Lauri, P., Di Fulvio, F., Forsell, N., Hasegawa, T., Krisztin, T., Palazzo, A., and Valin, H.: Land-based climate change mitigation potentials within the agenda for sustainable development, Environ. Res. Lett., 16, 024006, https://doi.org/10.1088/1748-9326/ABC58A, 2021.
Fricko, O., Parkinson, S. C., Johnson, N., Strubegger, M., Vliet, M. T. Van, and Riahi, K.: Energy sector water use implications of a 2° C climate policy, Environ. Res. Lett., 11, 034011, https://doi.org/10.1088/1748-9326/11/3/034011, 2016.
Frieler, K., Lange, S., Piontek, F., Reyer, C. P. O., Schewe, J., Warszawski, L., Zhao, F., Chini, L., Denvil, S., Emanuel, K., Geiger, T., Halladay, K., Hurtt, G., Mengel, M., Murakami, D., Ostberg, S., Popp, A., Riva, R., Stevanovic, M., Suzuki, T., Volkholz, J., Burke, E., Ciais, P., Ebi, K., Eddy, T. D., Elliott, J., Galbraith, E., Gosling, S. N., Hattermann, F., Hickler, T., Hinkel, J., Hof, C., Huber, V., Jägermeyr, J., Krysanova, V., Marcé, R., Müller Schmied, H., Mouratiadou, I., Pierson, D., Tittensor, D. P., Vautard, R., van Vliet, M., Biber, M. F., Betts, R. A., Bodirsky, B. L., Deryng, D., Frolking, S., Jones, C. D., Lotze, H. K., Lotze-Campen, H., Sahajpal, R., Thonicke, K., Tian, H., and Yamagata, Y.: Assessing the impacts of 1.5 °C global warming – simulation protocol of the Inter-Sectoral Impact Model Intercomparison Project (ISIMIP2b), Geosci. Model Dev., 10, 4321–4345, https://doi.org/10.5194/gmd-10-4321-2017, 2017.
Gernaat, D. E. H. J., de Boer, H. S., Daioglou, V., Yalew, S. G., Müller, C., and van Vuuren, D. P.: Climate change impacts on renewable energy supply, Nat. Clim. Change, 11, 119–125, https://doi.org/10.1038/s41558-020-00949-9, 2021.
Gleeson, T. and Richter, B.: How much groundwater can we pump and protect environmental flows through time? Presumptive standards for conjunctive management of aquifers and rivers, River Res. Appl., 34, 83–92, https://doi.org/10.1002/rra.3185, 2018.
Global Water Intelligence: DesalData, https://www.desaldata.com/ last access: 5 May 2023), 2016.
Graham, N. T., Hejazi, M. I., Chen, M., Davies, E. G. R., Edmonds, J. A., Kim, S. H., Turner, S. W. D., Li, X., Vernon, C. R., Calvin, K., Miralles-Wilhelm, F., Clarke, L., Kyle, P., Link, R., Patel, P., Snyder, A. C., and Wise, M. A.: Humans drive future water scarcity changes across all Shared Socioeconomic Pathways, Environ. Res. Lett., 15, 014007, https://doi.org/10.1088/1748-9326/AB639B, 2020.
Grubert, E. A.: Water consumption from hydroelectricity in the United States, Adv. Water Resour., 96, 88–94, https://doi.org/10.1016/J.ADVWATRES.2016.07.004, 2016.
Haddeland, I., Heinke, J., Biemans, H., Eisner, S., Flörke, M., Hanasaki, N., Konzmann, M., Ludwig, F., Masaki, Y., Schewe, J., Stacke, T., Tessler, Z. D., Wada, Y., and Wisser, D.: Global water resources affected by human interventions and climate change, P. Natl. Acad. Sci. USA, 111, 3251–3256, https://doi.org/10.1073/pnas.1308044111, 2014.
Harmsen, M., Kriegler, E., Van Vuuren, D. P., Van Der Wijst, K. I., Luderer, G., Cui, R., Dessens, O., Drouet, L., Emmerling, J., Morris, J. F., Fosse, F., Fragkiadakis, D., Fragkiadakis, K., Fragkos, P., Fricko, O., Fujimori, S., Gernaat, D., Guivarch, C., Iyer, G., Karkatsoulis, P., Keppo, I., Keramidas, K., Köberle, A., Kolp, P., Krey, V., Krüger, C., Leblanc, F., Mittal, S., Paltsev, S., Rochedo, P., Van Ruijven, B. J., Sands, R. D., Sano, F., Strefler, J., Arroyo, E. V., Wada, K., and Zakeri, B.: Integrated assessment model diagnostics: key indicators and model evolution, Environ. Res. Lett., 16, 054046, https://doi.org/10.1088/1748-9326/ABF964, 2021.
Havlík, P., Valin, H., Herrero, M., Obersteiner, M., Schmid, E., Rufino, M. C., Mosnier, A., Thornton, P. K., Böttcher, H., Conant, R. T., Frank, S., Fritz, S., Fuss, S., Kraxner, F., and Notenbaert, A.: Climate change mitigation through livestock system transitions, P. Natl. Acad. Sci. USA, 111, 3709–3714, https://doi.org/10.1007/s10640-017-0166-z, 2014.
Hejazi, M. I., Edmonds, J., Clarke, L., Kyle, P., Davies, E., Chaturvedi, V., Wise, M., Patel, P., Eom, J., and Calvin, K.: Integrated assessment of global water scarcity over the 21st century under multiple climate change mitigation policies, Hydrol. Earth Syst. Sci., 18, 2859–2883, https://doi.org/10.5194/hess-18-2859-2014, 2014.
Howard, P. H. and Sterner, T.: Few and Not So Far Between: A Meta-analysis of Climate Damage Estimates, Environ. Resour. Econ., 68, 197–225, https://doi.org/10.1007/s10640-017-0166-z, 2017.
Huppmann, D., Rogelj, J., Kriegler, E., Krey, V., and Riahi, K.: A new scenario resource for integrated 1.5 C research, Nat. Clim. Change, 8, 1027–1030, 2018.
Huppmann, D., Gidden, M., Fricko, O., Kolp, P., Orthofer, C., Pimmer, M., Kushin, N., Vinca, A., Mastrucci, A., Riahi, K., and Krey, V.: The MESSAGEix Integrated Assessment Model and the ix modeling platform (ixmp): An open framework for integrated and cross-cutting analysis of energy, climate, the environment, and sustainable development, Environ. Model. Softw., 143–156, https://doi.org/10.1016/j.envsoft.2018.11.012, 2019.
Jägermeyr, J., Müller, C., Ruane, A. C., Elliott, J., Balkovic, J., Castillo, O., Faye, B., Foster, I., Folberth, C., Franke, J. A., Fuchs, K., Guarin, J. R., Heinke, J., Hoogenboom, G., Iizumi, T., Jain, A. K., Kelly, D., Khabarov, N., Lange, S., Lin, T. S., Liu, W., Mialyk, O., Minoli, S., Moyer, E. J., Okada, M., Phillips, M., Porter, C., Rabin, S. S., Scheer, C., Schneider, J. M., Schyns, J. F., Skalsky, R., Smerald, A., Stella, T., Stephens, H., Webber, H., Zabel, F., and Rosenzweig, C.: Climate impacts on global agriculture emerge earlier in new generation of climate and crop models, Nat. Food, 2, 873–885, https://doi.org/10.1038/s43016-021-00400-y, 2021.
Jones, E. R., van Vliet, M. T. H., Qadir, M., and Bierkens, M. F. P.: Country-level and gridded estimates of wastewater production, collection, treatment and reuse, Earth Syst. Sci. Data, 13, 237–254, https://doi.org/10.5194/essd-13-237-2021, 2021.
Kapos Ravilious, C., Campbell, A., Dickson, B., Gibbs, H., Hansen, M., Lysenko, I., Miles, L., Price, J., Scharlemann, J. P. W., and Trumpe,r K.: Carbon and biodiversity, A demonstration atlas, UNEP-WCMC, Cambridge, UK, 2008.
Khan, Z., Linares, P., and García-González, J.: Integrating water and energy models for policy driven applications. A review of contemporary work and recommendations for future developments, Renewable and Sustainable Energy Reviews, 67, 1123–1138, https://doi.org/10.1016/j.rser.2016.08.043, 2017.
Khan, Z., Linares, P., Rutten, M., Parkinson, S., Johnson, N., and García-González, J.: Spatial and temporal synchronization of water and energy systems: Towards a single integrated optimization model for long-term resource planning, Appl. Energ., 210, 499–517, https://doi.org/10.1016/j.apenergy.2017.05.003, 2018.
Kikstra, J. S., Mastrucci, A., Min, J., Riahi, K., and Rao, N. D.: Decent living gaps and energy needs around the world, Environ. Res. Lett., 16, 095006, https://doi.org/10.1088/1748-9326/AC1C27, 2021.
Kim, S. H., Hejazi, M., Liu, L., Calvin, K., Clarke, L., Edmonds, J., Kyle, P., Patel, P., Wise, M., and Davies, E.: Balancing global water availability and use at basin scale in an integrated assessment model, Clim. Change, 136, 217–231, https://doi.org/10.1007/s10584-016-1604-6, 2016.
Kindermann, G. E., McCallum, I., Fritz, S., and Obersteiner, M.: A global forest growing stock, biomass and carbon map based on FAO statistics, Silva Fenn., 42, 387–396, https://doi.org/10.14214/SF.244, 2008.
Korkovelos, A., Khavari, B., Sahlberg, A., Howells, M., and Arderne, C.: The Role of Open Access Data in Geospatial Electrification Planning and the Achievement of SDG7. An OnSSET-Based Case Study for Malawi, Energies, 12, 1395, https://doi.org/10.3390/EN12071395, 2019.
Krey, V., Havlik, P., Kishimoto, P. N., Fricko, O., Zilliacus, J., Gidden, M., Strubegger, M., Kartasasmita, G., Ermolieva, T., Forsell, N., Gusti, M., Johnson, N., Kikstra, J., Kindermann, G., Kolp, P., Lovat, F., McCollum, D. L., Min, J., Pachauri, S., Parkinson, S. C., Rao, S., Rogelj, J., Ünlü, H., Valin, G., Wagner, P., Zakeri, B., Obersteiner, M., and Riahi, K.: MESSAGE-GLOBIOM 1.0 Documentation, https://doi.org/10.22022/iacc/03-2021.17115, 2016.
Lehner, B., Verdin, K., and Jarvis, A.: New global hydrography derived from spaceborne elevation data, Eos, Transactions, American Geophysical Union, 89, 93–94, https://doi.org/10.1029/2008eo100001, 2008.
Liu, Y., Hejazi, M., Kyle, P., Kim, S. H., Davies, E., Miralles, D. G., Teuling, A. J., He, Y., and Niyogi, D.: Global and regional evaluation of energy for water, Environ. Sci. Technol., 50, 9736–9745, https://doi.org/10.1021/ACS.EST.6B01065, 2016.
Mastrucci, A., Byers, E., Pachauri, S., and Rao, N. D.: Improving the SDG energy poverty targets: Residential cooling needs in the Global South, Energy Build, 186, 405–415, https://doi.org/10.1016/J.ENBUILD.2019.01.015, 2019.
Mastrucci, A., van Ruijven, B., Byers, E., Poblete-Cazenave, M., and Pachauri, S.: Global scenarios of residential heating and cooling energy demand and CO2 emissions, Clim. Change, 168, 1–26, https://doi.org/10.1007/s10584-021-03229-3, 2021.
Meinshausen, M., Raper, S. C. B., and Wigley, T. M. L.: Emulating coupled atmosphere-ocean and carbon cycle models with a simpler model, MAGICC6 – Part 1: Model description and calibration, Atmos. Chem. Phys., 11, 1417–1456, https://doi.org/10.5194/acp-11-1417-2011, 2011.
Meldrum, J., Nettles-Anderson, S., Heath, G., and Macknick, J.: Life cycle water use for electricity generation: a review and harmonization of literature estimates, Environ. Rese. Lett., 8, 015031, https://doi.org/10.1088/1748-9326/8/1/015031, 2013a.
Meldrum, J., Nettles-Anderson, S., Heath, G., and Macknick, J.: Life cycle water use for electricity generation: a review and harmonization of literature estimates, Environ. Res. Lett., 8, 015031, https://doi.org/10.1088/1748-9326/8/1/015031, 2013b.
Mohan, C., Western, A. W., Wei, Y., and Saft, M.: Predicting groundwater recharge for varying land cover and climate conditions – a global meta-study, Hydrol. Earth Syst. Sci., 22, 2689–2703, https://doi.org/10.5194/hess-22-2689-2018, 2018.
Müller, C. and Robertson, R. D.: Projecting future crop productivity for global economic modeling, Agr. Econ., 45, 37–50, https://doi.org/10.1111/AGEC.12088, 2014.
O'Neill, B. C., Kriegler, E., Ebi, K. L., Kemp-Benedict, E., Riahi, K., Rothman, D. S., van Ruijven, B. J., van Vuuren, D. P., Birkmann, J., Kok, K., Levy, M., and Solecki, W.: The roads ahead: Narratives for shared socioeconomic pathways describing world futures in the 21st century, Global Environ. Change, 42, 169–180, https://doi.org/10.1016/j.gloenvcha.2015.01.004, 2017.
Orthofer, C. L., Huppmann, D., and Krey, V.: South Africa after Paris-fracking its way to the NDCs?, Front. Energy Res., 7, 1–15, https://doi.org/10.3389/fenrg.2019.00020, 2019.
Pachauri, S., Poblete-Cazenave, M., Aktas, A., and Gidden, M. J.: Access to clean cooking services in energy and emission scenarios after COVID-19, Nature Energy, 6, 1067–1076, https://doi.org/10.1038/s41560-021-00911-9, 2021.
Palazzo, A., Valin, H., Batka, M., and Havlík, P.: Investment Needs for Irrigation Infrastructure along Different Socioeconomic Pathways, Investment Needs for Irrigation Infrastructure along Different Socioeconomic Pathways, Policy Research Working Paper, No. 8744, World Bank, Washington, DC., https://doi.org/10.1596/1813-9450-8744, 2019.
Parkinson, S., Krey, V., Huppmann, D., Kahil, T., McCollum, D., Fricko, O., Byers, E., Gidden, M. J., Mayor, B., Khan, Z., Raptis, C., Rao, N. D., Johnson, N., Wada, Y., Djilali, N., and Riahi, K.: Balancing clean water-climate change mitigation trade-offs, Environ. Res. Lett., 14, 014009, https://doi.org/10.1088/1748-9326/aaf2a3, 2019.
Parkinson, S. C., Makowski, M., Krey, V., Sedraoui, K., Almasoud, A. H., and Djilali, N.: A multi-criteria model analysis framework for assessing integrated water-energy system transformation pathways, Appl. Energ., 210, 477–486, https://doi.org/10.1016/j.apenergy.2016.12.142, 2018.
Parry, M. L. and Carter, T. R.: Climate impact assessment: A review of some approaches, Planning for Drought: Toward A Reduction of Societal Vulnerability, Planning for Drought, 165–187, https://doi.org/10.4324/9780429301735-13, 2019.
Pastor, A. V., Ludwig, F., Biemans, H., Hoff, H., and Kabat, P.: Accounting for environmental flow requirements in global water assessments, Hydrol. Earth Syst. Sci., 18, 5041–5059, https://doi.org/10.5194/hess-18-5041-2014, 2014.
Pastor, A. V., Palazzo, A., Havlik, P., Biemans, H., Wada, Y., Obersteiner, M., Kabat, P., and Ludwig, F.: The global nexus of food–trade–water sustaining environmental flows by 2050, Nat. Sustain., 2, 499–507, https://doi.org/10.1038/s41893-019-0287-1, 2019.
Patt, A. G., van Vuuren, D. P., Berkhout, F., Aaheim, A., Hof, A. F., Isaac, M., and Mechler, R.: Adaptation in integrated assessment modeling: Where do we stand?, Clim. Change, 99, 383–402, https://doi.org/10.1007/s10584-009-9687-y, 2010.
Piontek, F., Drouet, L., Emmerling, J., Kompas, T., Méjean, A., Otto, C., Rising, J., Soergel, B., Taconet, N., and Tavoni, M.: Integrated perspective on translating biophysical to economic impacts of climate change, Nat. Clim. Change, 11, 563–572, https://doi.org/10.1038/s41558-021-01065-y, 2021.
Platts Market Data – Electric Power | S&P Global Commodity Insights, https://www.spglobal.com/commodityinsights/en/products-services/electric-power/market-data-power, last access: 18 July 2022.
Poblete-Cazenave, M. and Pachauri, S.: A structural model of cooking fuel choices in developing countries, Energ. Econ., 75, 449–463, https://doi.org/10.1016/J.ENECO.2018.09.003, 2018.
Poblete-Cazenave, M. and Pachauri, S.: A model of energy poverty and access: Estimating household electricity demand and appliance ownership, Energ. Econ., 98, 105266, https://doi.org/10.1016/J.ENECO.2021.105266, 2021.
Poblete-Cazenave, M., Pachauri, S., Byers, E., Mastrucci, A., and van Ruijven, B.: Global scenarios of household access to modern energy services under climate mitigation policy, Nat. Energ., 6, 824–833, https://doi.org/10.1038/s41560-021-00871-0, 2021.
Prudhomme, C., Giuntoli, I., Robinson, E. L., Clark, D. B., Arnell, N. W., Dankers, R., Fekete, B. M., Franssen, W., Gerten, D., Gosling, S. N., Hagemann, S., Hannah, D. M., Kim, H., Masaki, Y., Satoh, Y., Stacke, T., Wada, Y., and Wisser, D.: Hydrological droughts in the 21st century, hotspots and uncertainties from a global multimodel ensemble experiment, P. Natl. Acad. Sci. USA, 111, 3262–3267, https://doi.org/10.1073/PNAS.1222473110, 2014.
Qadir, M., Drechsel, P., Jiménez Cisneros, B., Kim, Y., Pramanik, A., Mehta, P., and Olaniyan, O.: Global and regional potential of wastewater as a water, nutrient and energy source, Nat. Resour. Forum, 44, 40–51, https://doi.org/10.1111/1477-8947.12187, 2020.
Raptis, C. E., Van Vliet, M. T. H., and Pfister, S.: Global thermal pollution of rivers from thermoelectric power plants, Environ. Res. Lett., 11, 104011, https://doi.org/10.1088/1748-9326/11/10/104011, 2016.
Rasul, G.: Managing the food, water, and energy nexus for achieving the Sustainable Development Goals in South Asia, Environ. Dev., 18, 14–25, https://doi.org/10.1016/j.envdev.2015.12.001, 2016.
Rasul, G. and Sharma, B.: The nexus approach to water – energy – food security: an option for adaptation to climate change an option for adaptation to climate change, Clim. Policy, 16, 682–702, https://doi.org/10.1080/14693062.2015.1029865, 2016.
Riahi, K., van Vuuren, D. P., Kriegler, E., Edmonds, J., O'Neill, B. C., Fujimori, S., Bauer, N., Calvin, K., Dellink, R., Fricko, O., Lutz, W., Popp, A., Cuaresma, J. C., KC, S., Leimbach, M., Jiang, L., Kram, T., Rao, S., Emmerling, J., Ebi, K., Hasegawa, T., Havlik, P., Humpenöder, F., Da Silva, L. A., Smith, S., Stehfest, E., Bosetti, V., Eom, J., Gernaat, D., Masui, T., Rogelj, J., Strefler, J., Drouet, L., Krey, V., Luderer, G., Harmsen, M., Takahashi, K., Baumstark, L., Doelman, J. C., Kainuma, M., Klimont, Z., Marangoni, G., Lotze-Campen, H., Obersteiner, M., Tabeau, A., and Tavoni, M.: The Shared Socioeconomic Pathways and their energy, land use, and greenhouse gas emissions implications: An overview, Global Environ. Change, 42, 153–168, https://doi.org/10.1016/j.gloenvcha.2016.05.009, 2017.
Riahi, K., Bertram, C., Huppmann, D., Rogelj, J., Bosetti, V., Cabardos, A. M., Deppermann, A., Drouet, L., Frank, S., Fricko, O., Fujimori, S., Harmsen, M., Hasegawa, T., Krey, V., Luderer, G., Paroussos, L., Schaeffer, R., Weitzel, M., van der Zwaan, B., Vrontisi, Z., Longa, F. D., Després, J., Fosse, F., Fragkiadakis, K., Gusti, M., Humpenöder, F., Keramidas, K., Kishimoto, P., Kriegler, E., Meinshausen, M., Nogueira, L. P., Oshiro, K., Popp, A., Rochedo, P. R. R., Ünlü, G., van Ruijven, B., Takakura, J., Tavoni, M., van Vuuren, D., and Zakeri, B.: Cost and attainability of meeting stringent climate targets without overshoot, Nat. Clim. Change, 11, 1063–1069, https://doi.org/10.1038/s41558-021-01215-2, 2021.
Satoh, Y., Yoshimura, K., Pokhrel, Y., Kim, H., Shiogama, H., Yokohata, T., Hanasaki, N., Wada, Y., Burek, P., Byers, E., Schmied, H. M., Gerten, D., Ostberg, S., Gosling, S. N., Boulange, J. E. S., and Oki, T.: The timing of unprecedented hydrological drought under climate change, Nat. Commun., 13, 1–11, https://doi.org/10.1038/s41467-022-30729-2, 2022.
Schleussner, C.-F., Pfleiderer, P., Andrijevic, M., Vogel, M. M., Otto, F. E. L., and Seneviratne, S. I.: Pathways of climate resilience over the 21st century, Environ. Res. Lett., 16, 054058, https://doi.org/10.1088/1748-9326/abed79, 2021.
Schultes, A., Piontek, F., Soergel, B., Rogelj, J., Baumstark, L., Kriegler, E., Edenhofer, O., and Luderer, G.: Economic damages from on-going climate change imply deeper near-term emission cuts, Environ. Res. Lett., 16, 104053, https://doi.org/10.1088/1748-9326/AC27CE, 2021.
Soergel, B., Kriegler, E., Weindl, I., Rauner, S., Dirnaichner, A., Ruhe, C., Hofmann, M., Bauer, N., Bertram, C., Bodirsky, B. L., Leimbach, M., Leininger, J., Levesque, A., Luderer, G., Pehl, M., Wingens, C., Baumstark, L., Beier, F., Dietrich, J. P., Humpenöder, F., von Jeetze, P., Klein, D., Koch, J., Pietzcker, R., Strefler, J., Lotze-Campen, H., and Popp, A.: A sustainable development pathway for climate action within the UN 2030 Agenda, Nat. Clim. Change, 11, 656–664, https://doi.org/10.1038/s41558-021-01098-3, 2021.
Sutanudjaja, E. H., van Beek, R., Wanders, N., Wada, Y., Bosmans, J. H. C., Drost, N., van der Ent, R. J., de Graaf, I. E. M., Hoch, J. M., de Jong, K., Karssenberg, D., López López, P., Peßenteiner, S., Schmitz, O., Straatsma, M. W., Vannametee, E., Wisser, D., and Bierkens, M. F. P.: PCR-GLOBWB 2: a 5 arcmin global hydrological and water resources model, Geosci. Model Dev., 11, 2429–2453, https://doi.org/10.5194/gmd-11-2429-2018, 2018.
Tuninetti, M., Tamea, S., D'Odorico, P., Laio, F., and Ridolfi, L.: Global sensitivity of high-resolution estimates of crop water footprint, Water Resour. Res., 51, 8257–8272, https://doi.org/10.1002/2015WR017148, 2015.
Vinca, A., Parkinson, S., Byers, E., Burek, P., Khan, Z., Krey, V., Diuana, F. A., Wang, Y., Ilyas, A., Köberle, A. C., Staffell, I., Pfenninger, S., Muhammad, A., Rowe, A., Schaeffer, R., Rao, N. D., Wada, Y., Djilali, N., and Riahi, K.: The NExus Solutions Tool (NEST) v1.0: an open platform for optimizing multi-scale energy–water–land system transformations, Geosci. Model Dev., 13, 1095–1121, https://doi.org/10.5194/gmd-13-1095-2020, 2020.
van Maanen, N., Lissner, T., Harmsen, M., Piontek, F., Andrijevic, M., and van Vuuren, D. P.: Representation of adaptation in quantitative climate assessments, Nat. Clim. Change, 13, 309–311, https://doi.org/10.1038/s41558-023-01644-1, 2023.
van Vuuren, D. P., Edmonds, J., Kainuma, M., Riahi, K., Thomson, A., Hibbard, K., Hurtt, G. C., Kram, T., Krey, V., Lamarque, J. F., Masui, T., Meinshausen, M., Nakicenovic, N., Smith, S. J., and Rose, S. K.: The representative concentration pathways: An overview, Clim. Change, 109, 5–31, https://doi.org/10.1007/s10584-011-0148-z, 2011.
Wada, Y., Wisser, D., and Bierkens, M. F. P.: Global modeling of withdrawal, allocation and consumptive use of surface water and groundwater resources, Earth Syst. Dynam., 5, 15–40, https://doi.org/10.5194/esd-5-15-2014, 2014.
Wada, Y., Flörke, M., Hanasaki, N., Eisner, S., Fischer, G., Tramberend, S., Satoh, Y., van Vliet, M. T. H., Yillia, P., Ringler, C., Burek, P., and Wiberg, D.: Modeling global water use for the 21st century: the Water Futures and Solutions (WFaS) initiative and its approaches, Geosci. Model Dev., 9, 175–222, https://doi.org/10.5194/gmd-9-175-2016, 2016.
Wang, T. and Sun, F.: Global gridded GDP data set consistent with the shared socioeconomic pathways, Sci. Data, 9, 1–10, https://doi.org/10.1038/s41597-022-01300-x, 2022.
Wang, X., Zhang, J., Shahid, S., Guan, E., Wu, Y., Gao, J., and He, R.: Adaptation to climate change impacts on water demand, Mitig. Adapt. Strat. Gl., 21, 81–99, 2016.
Weyant, J.: Some contributions of integrated assessment models of global climate change, Rev. Environ. Econ. Policy, 11, 115–137, 2017.
Yalew, S. G., van Vliet, M. T. H., Gernaat, D. E. H. J., Ludwig, F., Miara, A., Park, C., Byers, E., De Cian, E., Piontek, F., Iyer, G., Mouratiadou, I., Glynn, J., Hejazi, M., Dessens, O., Rochedo, P., Pietzcker, R., Schaeffer, R., Fujimori, S., Dasgupta, S., Mima, S., da Silva, S. R. S., Chaturvedi, V., Vautard, R., and van Vuuren, D. P.: Impacts of climate change on energy systems in global and regional scenarios, Nat. Energ., 5, 794–802, https://doi.org/10.1038/s41560-020-0664-z, 2020.
Yates, D. N.: Approaches to continental scale runoff for integrated assessment models, J. Hydrol., 201, 289–310, https://doi.org/10.1016/S0022-1694(97)00044-9, 1997.