the Creative Commons Attribution 4.0 License.
the Creative Commons Attribution 4.0 License.
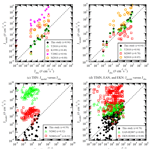
H2SO4–H2O binary and H2SO4–H2O–NH3 ternary homogeneous and ion-mediated nucleation: lookup tables version 1.0 for 3-D modeling application
Alexey B. Nadykto
Jason Herb
Formation of new particles in the atmosphere has important implications for air quality and climate. Recently, we have developed a kinetically based H2SO4–H2O–NH3-ion nucleation model which well captures the absolute values of nucleation rates as well as dependencies of nucleation rates on NH3 and H2SO4 concentrations, ionization rates, temperature, and relative humidity observed in the well-controlled Cosmics Leaving Outdoor Droplets (CLOUD) measurements. Here we employ the aforementioned recently developed kinetic nucleation model to generate nucleation rate lookup tables for H2SO4–H2O binary homogenous nucleation (BHN), H2SO4–H2O–NH3 ternary homogeneous nucleation (THN), H2SO4–H2O-ion binary ion-mediated nucleation (BIMN), and H2SO4–H2O–NH3-ion ternary ion-mediated nucleation (TIMN). A comparison of nucleation rates calculated using the lookup tables with CLOUD measurements of BHN, BIMN, THN, and TIMN is presented. The lookup tables cover a wide range of key parameters controlling binary, ternary, and ion-mediated nucleation in the Earth's atmosphere and are a cost-efficient solution for multidimensional modeling. The lookup tables and FORTRAN codes, made available through this work, can be readily used in 3-D modeling. The lookup tables can also be used by experimentalists involved in laboratory and field measurements for a quick assessment of nucleation involving H2SO4, H2O, NH3, and ions.
Particles in the troposphere either come from direct emission (i.e., primary particles) or in situ nucleation (i.e., secondary particles). Secondary particles formed via nucleation dominate the number of concentrations of atmospheric particles (Spracklen et al., 2008; Pierce and Adams, 2009; Yu and Luo, 2009) that are important for air quality and climate. Nucleation in the atmosphere is a dynamic process involving various interactions of precursor gas molecules, small clusters, and pre-existing particles (Yu and Turco, 2001; R. Zhang et al., 2012; Lee et al., 2019). H2SO4 and H2O are known to play an important role in atmospheric new-particle formation (NPF; e.g., Doyle, 1961). It has been long known that while binary homogeneous nucleation (BHN) of H2SO4–H2O may play a dominant role in the cold upper troposphere, it cannot explain nucleation events observed in the lower troposphere (e.g., Weber et al., 1996). Several alternative nucleation theories have been proposed, including ternary homogeneous nucleation (THN) involving NH3 (Coffman and Hegg, 1995; Napari et al., 2002), ion-mediated nucleation (IMN) considering the role of the ubiquitous ion in enhancing the stability and growth of prenucleation clusters (Yu and Turco, 2001), and nucleation involving organic compounds (e.g., Zhang et al., 2004). The laboratory measurements in the CLOUD (Cosmics Leaving Outdoor Droplets) chamber experiments at CERN show that both ammonia and ionization can enhance H2SO4–H2O nucleation (Kirkby et al., 2011). In order to reach a deep and insightful understanding of the physicochemical processes underlying the observed enhancement effect of ammonia and ions, Yu et al. (2018) developed a kinetic ternary ion-mediated nucleation (TIMN) model for the H2SO4–H2O–NH3-ion system with thermodynamic data derived from laboratory measurements and quantum chemical calculations. The model is able to explain the observed difference in the effect of NH3 in lowering the nucleation barriers for clusters of different charging states and predicts nucleation rates in good agreement with CLOUD observations (Yu et al., 2018).
The main objective of this work is to employ the recently developed kinetic nucleation model (Yu et al., 2018) to generate nucleation rate lookup tables for four different nucleation pathways: H2SO4–H2O binary homogenous nucleation (BHN), H2SO4–H2O–NH3 ternary homogeneous nucleation (THN), H2SO4–H2O-ion binary ion-mediated nucleation (BIMN), and H2SO4–H2O–NH3-ion ternary ion-mediated nucleation (TIMN). With the lookup tables and simple interpolation subroutines, the computational costs of the binary and ternary nucleation rate calculations were significantly reduced, which is critically important for multidimensional modeling. The computed nucleation rates of BHN, THN, BIMN, and TIMN based on the lookup tables were evaluated against CLOUD measurements.
The H2SO4–H2O–NH3-ion kinetic nucleation model, as described in detail in Yu et al. (2018) solves the dynamic interactions of various clusters and offers a physics-based explanation of the different concentrations of NH3 needed to induce nucleation on neutral clusters, positive ions, and negative ions. The model is designed for a nucleating system consisting of H2SO4–H2O–NH3 in the presence of ionization (i.e., ternary ion-mediated nucleation, TIMN). In the absence of NH3, the model transforms into binary homogeneous nucleation (BHN) or binary ion-mediated nucleation (BIMN) and reduces to BHN or ternary homogeneous nucleation (THN) in the case when no ions are present. It is important to note that in the H2SO4–H2O–NH3 ternary system, binary H2SO4–H2O clusters coexist with ternary H2SO4–H2O–NH3 ones, while in the system with ions, neutral clusters coexist with charged clusters. Therefore, BIMN includes BHN, THN includes BHN, and TIMN includes both BIMN and THN.
For the benefit of different applications and for enabling one to evaluate the contribution of different nucleation pathways (binary versus ternary, neural versus ion-mediated), we run the model to generate nucleation lookup tables separately for the four different nucleating systems, i.e., H2SO4–H2O (BHN), H2SO4–H2O–NH3 (THN), H2SO4–H2O-ion (BIMN), and H2SO4–H2O–NH3-ion (TIMN). One can accurately determine the role of NH3 by looking into the difference between BHN (BIMN) and THN (TIMN) rates and the role of ionization by examining the difference between BHN (THN) and BIMN (TIMN) rates. Another benefit of generating separate lookup tables is that for the users who are only interested in BHN, BIMN, or THN, the corresponding lookup tables are much smaller than that of TIMN and much easier to handle.
For many practical applications, steady-state nucleation rates under given conditions are required. Nucleation rates are conventionally calculated at the sizes of critical clusters (Seinfeld and Pandis, 2016). Since the kinetic nucleation model explicitly solves the evolution of clusters of various sizes, it can calculate steady-state particle formation rates at any sizes larger than critical sizes (Yu, 2006). In many laboratory studies new-particle formation rates have been measured at certain detection sizes, typically much larger than critical sizes. For example, the nucleation rates measured in the CLOUD experiment are for particles with a mobility diameter of 1.7 nm. For atmospheric modeling with size-resolved particle microphysics, the sizes of the first bin are generally much larger than the critical sizes, and the nucleation rates calculated at the critical sizes (which vary with the atmospheric conditions) have to be extrapolated to the sizes of the first bin based on the assumed growth rates and coagulation sinks of freshly nucleated particles that may lead to additional uncertainties. To compare model nucleation rates with typical laboratory measurements and to facilitate the application of the obtained results in a size-resolved particle microphysics model whose first bin can have a size of down to around 1–2 nm. Nucleation rates are calculated at 1.7 nm mobility diameter (corresponding to mass diameter of ∼1.5 nm; Yu et al., 2018).
Table 1The range of values for each independent variable in the BHN, THN, BIMN, and TIMN nucleation rate lookup tables. Also given are the total number of values for each variable, the specific values at which nucleation rates have been calculated, and controlling parameters for the four nucleation mechanisms.
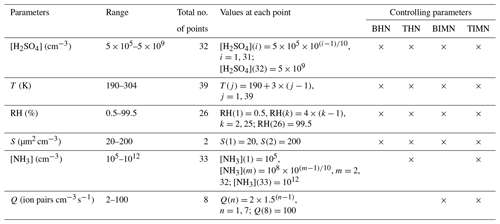
Lookup tables of steady-state nucleation rates for BHN (JBHN), THN (JTHN), BIMN (JBIMN), and TIMN (JTIMN) have been generated under a wide range of atmospheric conditions. There are six parameters controlling JTIMN: sulfuric acid vapor concentration ([H2SO4]), ammonia gas concentration ([NH3]), temperature (T), relative humidity (RH), ionization rate (Q), and surface area of pre-existing particles (S). Compared to JTIMN, there is one fewer controlling parameter for both JBIMN (no [NH3] dependence) and JTHN (no Q dependence), while JBHN only depends on four parameters ([H2SO4] T, RH, and S). Table 1 gives the range of each dependent variable dimension, total number of points in each dimension, values at each point, and controlling parameters for the four nucleation pathways. The range and resolution in each parameter space are designed based on the sensitivity of nucleation rates to the parameter, its possible range in the troposphere, and a balance between the accuracy and sizes of the lookup tables. T ranges from 190 to 304 K (resolution: 3 K), and RH (with respect to water) ranges from 0.5 % to 99.5 % (resolution: 4 %). For [H2SO4], we use 31 points to cover 5×105 to 5×108 cm−3 plus one additional point at cm−3. For [NH3], we employ 31 points to cover 108 to 1011 cm−3 plus two additional points at [NH3]=105 and 1012 cm−3. Q ranges from 2 to 23 ion pairs with the resolution of five values per decade (geometric) plus one additional point at Q=100 ion pairs (noting that Q=0 ion pairs is covered under BHN or THN). S ranges from 20 to 200 µm2 cm−3 with two points. Almost all the possible tropospheric conditions relevant to NPF shall be covered with the above parameter ranges. The lookup tables are designed to calculate nucleation rates in the troposphere. For conditions in the stratosphere (RH<0.5 %) and on other planets (such as on Venus, as discussed in Määttänen et al., 2018), it is unclear whether the model is valid or not as measurements under such conditions are not available to validate the model.
The lookup table for JTIMN is the largest, being composed of JTIMN at more than 17 million points () and with a total text format size of ∼103 MB. For comparison, the smallest lookup table (for JBHN) has just 64 896 points and a total text format size of ∼0.38 MB. For any given values of [H2SO4], [NH3], T, RH, Q, and S within the ranges specified in Table 1, nucleation rates can be obtained using the lookup tables with an efficient multiple-variable interpolation scheme as described in Yu (2010). For conditions out of the ranges specified in Table 1, which may occur occasionally in the atmosphere, linear extrapolation is allowed only for surface area, for which the tables only give values at two surface area points (S=20 and 200 µm2 cm−3). The dependence of nucleation rates on the surface area, which serves as a coagulation sink (not a condensation sink because [H2SO4] is fixed), is relatively linear, and thus extrapolation (linearly between Log10J versus surface area) will not cause unphysical values. The JBHN, JTHN, JBIMN, and JTIMN lookup tables can be accessed via the information given in the data availability section and can be used to calculate nucleation rates efficiently in 3-D models.
Compared to those based on the full model, the deviation of nucleation rates based on the lookup tables is generally within a factor of 2, well within the corresponding uncertainty of CLOUD measurements. The dependence of nucleation rates on the surface area is relatively linear, and two points for S provide reasonable accuracy (compared to the uncertainties in the model itself and measurements). In the atmosphere, the surface area of pre-existing particles not only serves as a coagulation sink but also as a condensation sink for H2SO4, and thus it has a more profound impact because nucleation rates are highly sensitive to [H2SO4]. For the lookup tables, [H2SO4] is fixed, and therefore the dependence of nucleation rates on surface area is relatively weaker. It should be noted that most existing nucleation parameterizations do not take into account the effect of surface area.
Dunne et al. (2016) reported CLOUD-measured nucleation rates under 377 different conditions (Table S1 of Dunne et al., 2016). These data can be divided into BHN, THN, BIMN, and TIMN based on the values of [NH3] and Q in the chamber. Nucleation is classified as neutral (BHN or THN) when Q=0 and as binary (BHN or BIMN) when [NH3]<0.1 ppt. As a result, 15, 27, 110, and 225 of these CLOUD measurements correspond to BHN, BIMN, THN, and TIMN, respectively. Figures 1–4 present the comparisons of the nucleation rates calculated from the lookup tables (Jmodel) with corresponding values observed during CLOUD experiments (Jobs) under BHN, BIMN, THN, and TIMN conditions. The error bars give the Jmodel range as a result of the measured [H2SO4] uncertainty (−50 %, +100 %). The uncertainties in Jobs (overall a factor of 2) and those associated with the uncertainty in measured [NH3] (−50 %, +100 %) are not included.
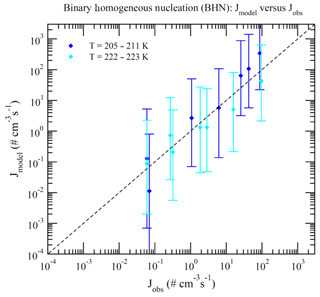
Figure 1Model-predicted (Jmodel) versus observed (Jobs) nucleation rates under BHN conditions (no ionization, [NH3]<0.1 ppt) of CLOUD measurements reported in Table S1 of Dunne et al. (2016). The data points are grouped according to temperatures as specified in the legend. Vertical error bars show the range of Jmodel calculated at 50 % and 200 % of measured [H2SO4], corresponding to the uncertainties in measured [H2SO4] (−50 %, +100 %). Error bars associated with the uncertainties in measured [NH3] (−50 %, +100 %) and Jobs (overall a factor of 2) are not shown.
Because of the increase in the contamination (both unwanted ammonia and amines) with the CLOUD chamber temperature (Kürten et al., 2016), binary nucleation measurements (i.e., without ammonia, [NH3]<0.1 ppt) are only available at very low T (Figs. 1–2). Both BHN and BIMN predictions based on the lookup tables overall agree well with the available CLOUD observations within the uncertainty range. As pointed out earlier, binary H2SO4–H2O clusters coexist with ternary H2SO4–H2O–NH3 ones in the ternary system, while neutral clusters coexist with charged clusters in the system containing ions. Therefore, the nice agreement of BHN and BIMN model predictions with observations provides a good foundation for the more complex THN and TIMN models. CLOUD experiments have more data points for THN and TIMN within a wide temperature range covering the lower troposphere. For THN (Fig. 3), the model prediction is consistent with measurements at a low temperature (–250 K) but deviates from measurements at high T, with the level of model underprediction increasing with increasing T. As pointed out in Yu et al. (2018), the level of contamination in the CLOUD chamber appears to increase with temperature (Kürten et al., 2016); the nice agreement at lower T and the deviation at higher T may be associated with contamination (such as amines, etc.) in the CLOUD (Kirkby et al., 2011) that increases with temperature (Kürten et al., 2016). In contrast to THN, Jmodel for TIMN (Fig. 4) agrees with CLOUD measurements within the uncertainties under nearly all conditions. Jmodel for TIMN at T=292–300 K is slightly lower than the corresponding observed values, which is likely a result of similar causes of the THN underprediction at higher T (Fig. 3). As demonstrated in Yu et al. (2018), the nucleation of ions is typically stronger than that of neutral clusters for both binary and ternary nucleating systems with ammonia. The ubiquitous presence of ionization in the Earth's atmosphere calls for regional and global aerosol models to take into account the effect of ionization in NPF. The BIMN and TIMN lookup tables, derived from a physics-based kinetic nucleation model and validated against the state-of-the-art CLOUD measurements, provide an efficient way to incorporate the role of ionization in new particle formation in 3-D models.
Many global models explicitly calculate nucleation rates, but different models and studies employ quite different nucleation schemes (e.g., Wang and Penner, 2009; Zhang et al., 2010; Yu et al., 2010, 2012; Liu et al., 2012; K. Zhang et al., 2012; Williamson et al., 2019). For example, the BHN scheme of Vehkamäki et al. (2002; hereafter V2002) was used by the CAM5 (Liu et al., 2012) and ECHAM5-HAM (Stier et al., 2005) models. The H2SO4–H2O ion-induced nucleation (IIN, similar to BIMN defined in this study) of Lovejoy et al. (2004) and Kazil and Lovejoy (2007) was considered in the ECHAM5-HAM2 model (Kazil et al., 2010; K. Zhang et al., 2012). The H2SO4–H2O ion-mediated nucleation scheme of Yu and Turco (2001) and Yu (2010) was employed by the GEOS-Chem (Yu et al., 2010) and CAM5 (Yu et al., 2012) models. In addition, some aerosol models (Wang and Penner, 2009; K. Zhang et al., 2012) used the empirical nucleation parameterization for the boundary layer (e.g., Kuang et al., 2008) in combination with the binary nucleation scheme. It is of interest to understand the differences in nucleation rates predicted by different nucleation schemes under the well-controlled CLOUD conditions.
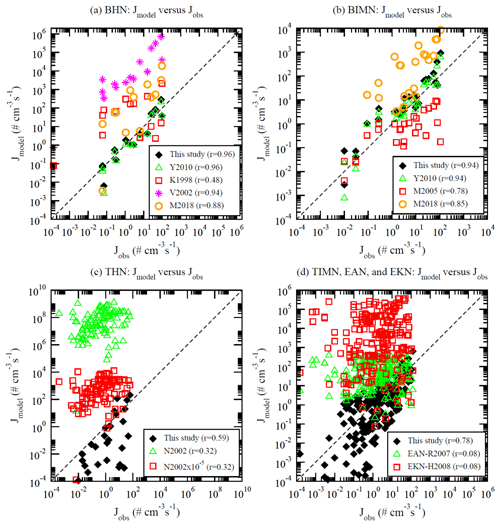
Figure 5Comparison of nucleation rates based on different models and parameterizations (Jmodel) versus corresponding observed values (Jobs) under (a) BHN, (b) BIMN, (c) THN, and (d) TIMN conditions of CLOUD measurements reported in Table S1 of Dunne et al. (2016). See the text for the references of the models and parameterizations considered here. The correlation coefficient (r) between log10(Jmodel) based on each scheme and log10(Jobs) is given in the figure legend. The dashed line shows the 1:1 ratio.
Figure 5 compares nucleation rates calculated based on lookup tables presented in this work and several other aerosol nucleation parameterizations with the CLOUD measurements. The nucleation models and parameterizations considered in Fig. 5 include this study (i.e., the lookup tables described in this paper); BHN of Kulmala et al. (1998; hereafter K1998) and Vehkamäki et al. (2002; hereafter V2002); BHN and BIMN of Yu (2010; hereafter Y2010) and Määttänen et al. (2018; hereafter M2018); IIN (same as the BIMN) of Modgil et al. (2005; hereafter M2005), which is a parameterization based on Lovejoy et al. (2004); THN of Napari et al. (2002; hereafter N2002); empirical activation nucleation (EAN) parameterization of Riipinen et al. (2007; hereafter EAN-R2007; [H2SO4]); and empirical kinetic nucleation (EKN) parameterization of Kuang et al. (2008; hereafter EKN-H2008; [H2SO4]2). The EAN and EKN parameterizations were derived from atmospheric nucleation measurements in the boundary layer (with the presence of ammonia and ionization) and thus are compared with the TIMN scheme (Fig. 5d). For THN (Fig. 5c), N2002 scaled by 10−5 has been used in some modeling studies (e.g., Williamson et al., 2019), and thus values of N2002 are also given in Fig. 5c for comparisons. It can be seen from Fig. 5 that there exist large differences in the nucleation rates predicted by different nucleation schemes and parameterizations, and the CLOUD measurements provide useful constraints to the nucleation schemes. Among the schemes considered in Fig. 5, the lookup tables presented in this work are in the best agreement with CLOUD measurements for all four nucleation pathways in terms of not only the absolute nucleation rates but also the correlation coefficients. BHN rates based on K2008, V2002, and M2018 are generally 1–4 orders of magnitude higher than the observed values, with K1998 having the lowest correlation coefficient (r=0.48). For BIMN, M2005 generally underpredicts while M2008 overestimates the rates by up to ∼2 orders of magnitude. For THN, N2002 significantly overestimates the rates by 5–9 orders of magnitude. The scaling of N2002 by 10−5 reduces the overestimation, but the correlation coefficient remains low (r=0.32). The empirical parameterizations (both EAN and EKN) depend only on [H2SO4] and, unsurprisingly, have very low correlation coefficients (r=0.08) with CLOUD measurements. Care should be taken in employing the empirical parameterizations in global models as both EAN and EKN may give incorrect spatial distributions (Yu et al., 2010) and temporal variations of nucleation rates in the atmosphere. It should be noted that the TIMN scheme can be directly applied to calculate nucleation rates in the whole troposphere (including the boundary layer), and thus one shall not combine the BHN, THN, BIMN, and TIMN schemes presented in this study with empirical boundary nucleation parameterizations (i.e., EAN and EKN) in regional and global simulations.
The code and lookup tables can be accessed via the zenodo data repository (https://doi.org/10.5281/zenodo.3483797; Yu, 2019). For quick calculation of BHN, THN, BIMN, and TIMN rates under specified conditions, one can use the online nucleation calculators, which we have developed based on these lookup tables and made available to the public at http://apm.asrc.albany.edu/nrc/ (last access: 15 June 2020).
FY designed and generated the lookup tables. FY, ABN, GL, and JH contributed to the kinetic model used to generate the lookup tables. FY wrote the paper with contributions from all coauthors.
The authors declare that they have no conflict of interest.
This research has been supported by the US National Science Foundation (grant no. AGS1550816) and the Russian Science Foundation and the Ministry of Science and Education of Russia (grant nos. 1.6198.2017/6.7 and 1.7706.2017/8.9).
This paper was edited by Samuel Remy and reviewed by three anonymous referees.
Coffman, D. J. and Hegg, D. A.: A preliminary study of the effect of ammonia on particle nucleation in the marine boundary layer, J. Geophys. Res., 100, 7147–7160, 1995.
Doyle, G. J.: Self-nucleation in the sulfuric acid-water system, J. Chem. Phys., 35, 795–799, 1961.
Dunne, E. M., Gordon, H., Kürten, A., Almeida, J., Duplissy, J., Williamson, C., Ortega, I. K., Pringle, K. J., Adamov, A., Baltensperger, U., Barmet, P., Benduhn, F., Bianchi, F., Breitenlechner, M., Clarke, A., Curtius, J., Dommen, J., Donahue, N. M., Ehrhart, S., Flagan, R. C., Franchin, A., Guida, R., Hakala, J., Hansel, A., Heinritzi, M., Jokinen, T., Kangasluoma, J., Kirkby, J., Kulmala, M., Kupc, A., Lawler, M. J., Lehtipalo, K., Makhmutov, V., Mann, G., Mathot, S., Merikanto, J., Miettinen, P., Nenes, A., Onnela, A., Rap, A., Reddington, C. L. S., Riccobono, F., Richards, N. A. D., Rissanen, M. P., Rondo, L., Sarnela, N., Schobesberger, S., Sengupta, K., Simon, M., Sipilä, M., Smith, J. N., Stozkhov, Y., Tomé, A., Tröstl, J., Wagner, P. E., Wimmer, D., Winkler, P. M., Worsnop, D. R., and Carslaw, K. S.: Global particle formation from CERN CLOUD measurements, Science, 354, 1119–1124, https://doi.org/10.1126/science.aaf2649, 2016.
Kazil, J. and Lovejoy, E. R.: A semi-analytical method for calculating rates of new sulfate aerosol formation from the gas phase, Atmos. Chem. Phys., 7, 3447–3459, https://doi.org/10.5194/acp-7-3447-2007, 2007.
Kazil, J., Stier, P., Zhang, K., Quaas, J., Kinne, S., O'Donnell, D., Rast, S., Esch, M., Ferrachat, S., Lohmann, U., and Feichter, J.: Aerosol nucleation and its role for clouds and Earth's radiative forcing in the aerosol-climate model ECHAM5-HAM, Atmos. Chem. Phys., 10, 10733–10752, https://doi.org/10.5194/acp-10-10733-2010, 2010.
Kirkby, J., Curtius, J., Almeida, J., Dunne, E., Duplissy, J., Ehrhart, S., Franchin, A., Gagné, S., Ickes, L., Kürten, A., Kupc, A., Metzger, A., Riccobono, F., Rondo, L., Schobesberger, S., Tsagkogeorgas, G., Wimmer, D., Amorim, A., Bianchi, F., Breitenlechner, M., David, A., Dommen, J., Downard, A., Ehn, M., Flagan, R. C., Haider, S., Hansel, A., Hauser, D., Jud,W., Junninen, H., Kreissl, F., Kvashin, A., Laaksonen, A., Lehtipalo, K., Lima, J., Lovejoy, E. R., Makhmutov, V., Mathot, S., Mikkilä, J., Minginette, P., Mogo, S., Nieminen, T., Onnela, A., Pereira, P., Petäjä, T., Schnitzhofer, R., Seinfeld, J. H., Sipilä, M., Stozhkov, Y., Stratmann, F., Tomé, A., Vanhanen, J., Viisanen, Y., Vrtala, A., Wagner, P. E., Walther, H., Weingartner, E., Wex, H., Winkler, P. M., Carslaw, K. S., Worsnop, D. R., Baltensperger, U., and Kulmala, M.: The role of sulfuric acid, ammonia and galactic cosmic rays in atmospheric aerosol nucleation, Nature, 476, 429–433, 2011.
Kuang, C., McMurry, P. H., McCormick, A. V., and Eisele, F. L.: Dependence of nucleation rates on sulfuric acid vapor concentration in diverse atmospheric locations, J. Geophys. Res., 113, D10209, https://doi.org/10.1029/2007JD009253, 2008.
Kulmala, M., Laaksonen, A., and Pirjola, L.: Parameterizations for sulfuric acid/water nucleation rates, J. Geophys. Res., 103, 8301–8308, 1998.
Kürten, A., Bianchi, F., Almeida, J., Kupiainen-Määttä, O., Dunne, E. M., Duplissy, J., Williamson, C., Barmet, P., Breitenlechner, M., Dommen, J., Donahue, N. M., Flagan, R. C., Franchin, A., Gordon, H., Hakala, J., Hansel, A., Heinritzi, M., Ickes, L., Jokinen, T., Kangasluoma, J., Kim, J., Kirkby, J., Kupc, A., Lehtipalo, K., Leiminger, M., Makhmutov, V., Onnela, A., Ortega, I. K., Petäjä, T., Praplan, A. P., Riccobono, F., Rissanen, M. P., Rondo, L., Schnitzhofer, R., Schobesberger, S., Smith, J. N., Steiner, G., Stozhkov, Y., Tomé, A., Tröstl, J., Tsagkogeorgas, G., Wagner, P. E., Wimmer, D., Ye, P., Baltensperger, U., Carslaw, K., Kulmala, M., and Curtius, J.: Experimental particle formation rates spanning tropospheric sulfuric acid and ammonia abundances, ion production rates, and temperatures, J. Geophys. Res.-Atmos., 121, 12377–12400, https://doi.org/10.1002/2015JD023908, 2016.
Lee, S.-H., Gordon, H., Yu, H., Lehtipalo, K., Haley, R., Li, Y., and Zhang, R.: New particle formation in the atmosphere: From molecular clusters to global climate, J. Geophys. Res., 124, 7098–7146, https://doi.org/10.1029/2018JD029356, 2019.
Liu, X., Easter, R. C., Ghan, S. J., Zaveri, R., Rasch, P., Shi, X., Lamarque, J.-F., Gettelman, A., Morrison, H., Vitt, F., Conley, A., Park, S., Neale, R., Hannay, C., Ekman, A. M. L., Hess, P., Mahowald, N., Collins, W., Iacono, M. J., Bretherton, C. S., Flanner, M. G., and Mitchell, D.: Toward a minimal representation of aerosols in climate models: description and evaluation in the Community Atmosphere Model CAM5, Geosci. Model Dev., 5, 709–739, https://doi.org/10.5194/gmd-5-709-2012, 2012.
Lovejoy, E. R., Curtius, J., and Froyd, K. D.: Atmospheric ion-induced nucleation of sulfuric acid and water, J. Geophys. Res., 109, D08204, https://doi.org/10.1029/2003JD004460, 2004.
Määttänen, A., Merikanto, J., Henschel, H., Duplissy, J., Makkonen, R., Ortega, I. K., and Vehkamäki, H.: New Parameterizations for Neutral and Ion-Induced Sulfuric Acid-Water Particle Formation in Nucleation and Kinetic Regimes, J. Geophys. Res.-Atmos., 123, 1269–1296. https://doi.org/10.1002/2017JD027429, 2018.
Modgil, M. S., Kumar, S., Tripathi, S. N., and Lovejoy, E. R.: A parameterization of ion-induced nucleation of sulphuric acid and water for atmospheric conditions, J. Geophys. Res., 110, D19205, https://doi.org/10.1029/2004JD005475, 2005.
Napari, I., Noppel, M., Vehkamaki, H., and Kulmala, M., Parameterization of Ternary Nucleation Rates for H2SO4-NH3-H2O Vapors, J. Geophys. Res., 107, 4381, https://doi.org/10.1029/2002JD002132, 2002.
Pierce, J. R. and Adams, P. J.: Uncertainty in global CCN concentrations from uncertain aerosol nucleation and primary emission rates, Atmos. Chem. Phys., 9, 1339–1356, https://doi.org/10.5194/acp-9-1339-2009, 2009.
Riipinen, I., Sihto, S.-L., Kulmala, M., Arnold, F., Dal Maso, M., Birmili, W., Saarnio, K., Teinilä, K., Kerminen, V.-M., Laaksonen, A., and Lehtinen, K. E. J.: Connections between atmospheric sulphuric acid and new particle formation during QUEST III–IV campaigns in Heidelberg and Hyytiälä, Atmos. Chem. Phys., 7, 1899–1914, https://doi.org/10.5194/acp-7-1899-2007, 2007.
Seinfeld, J. H. and Pandis, S. N.: Atmospheric Chemistry and Physics From Air Pollution to Climate Change. John Wiley & Sons, Hoboken, 2016.
Spracklen, D. V., Carslaw, K. S., Kulmala, M., Kerminen, V.-M., Sihto, Riipinen, I., Merikanto, J., Mann, G. W., Chipperfield, M. P., Wiedensohler, A., Birmili, W., and Lihavainen, H.: Contribution of particle formation to global cloud condensation nuclei concentrations, Geophys. Res. Lett., 35, L06808, https://doi.org/10.1029/2007GL033038, 2008.
Stier, P., Feichter, J., Kinne, S., Kloster, S., Vignati, E., Wilson, J., Ganzeveld, L., Tegen, I., Werner, M., Balkanski, Y., Schulz, M., Boucher, O., Minikin, A., and Petzold, A.: The aerosol-climate model ECHAM5-HAM, Atmos. Chem. Phys., 5, 1125–1156, https://doi.org/10.5194/acp-5-1125-2005, 2005.
Vehkamäki, H., Kulmala, M., Napari, I., Lehtinen, K. E. J., Timmreck, C., Noppel, M., and Laaksonen, A.: An improved parameterization for sulfuric acid–water nucleation rates for tropospheric and stratospheric conditions, J. Geophys. Res., 107, 4622, https://doi.org/10.1029/2002JD002184, 2002.
Wang, M. and Penner, J. E.: Aerosol indirect forcing in a global model with particle nucleation, Atmos. Chem. Phys., 9, 239–260, https://doi.org/10.5194/acp-9-239-2009, 2009.
Weber, R. J., Marti, J. J., McMurry, P. H., Eisele, F. L., Tanner, D. J., and Jefferson, A.: Measured atmo-spheric new particle formation rates: implications for nu-cleation mechanisms, Chem. Eng. Commun., 151, 53–64, https://doi.org/10.1080/00986449608936541, 1996.
Williamson, C. J., Kupc, A., Axisa, D., Bilsback, K. R., Bui, T., Campuzano-Jost, P., Dollner, M., Froyd, K. D., Hodshire, A. L., Jimenez, J. L., Kodros, J. K., Luo, G., Murphy, D. M., Nault, B. A., Ray, E. A., Weinzierl, B., Wilson, J. C., Yu, F., Yu, P., Pierce, J. R., and Brock, C. A.: A Large Source of Cloud Condensation Nuclei from New Particle Formation in the Tropics, Nature, 574, 399–403, 2019.
Yu, F.: From molecular clusters to nanoparticles: second-generation ion-mediated nucleation model, Atmos. Chem. Phys., 6, 5193–5211, https://doi.org/10.5194/acp-6-5193-2006, 2006.
Yu, F.: Ion-mediated nucleation in the atmosphere: Key controlling parameters, implications, and look-up table, J. Geophy. Res., 115, D03206, https://doi.org/10.1029/2009JD012630, 2010.
Yu, F.: Lookup tables for H2SO4-H2O binary and H2SO4-H2O-NH3 ternary homogeneous and ion-mediated nucleation (Version 1.0.0) [Data set], Zenodo, https://doi.org/10.5281/zenodo.3483797, 2019.
Yu, F. and Luo, G.: Simulation of particle size distribution with a global aerosol model: contribution of nucleation to aerosol and CCN number concentrations, Atmos. Chem. Phys., 9, 7691–7710, https://doi.org/10.5194/acp-9-7691-2009, 2009.
Yu, F. and Turco, R. P.: From molecular clusters to nanoparticles: The role of ambient ionization in tropospheric aerosol formation, J. Geophys. Res., 106, 4797–4814, 2001.
Yu, F., Luo, G., Bates, T., Anderson, B., Clarke, A., Kapustin, V., Yantosca, R., Wang, Y., and Wu, S.: Spatial distributions of particle number concentrations in the global troposphere: Simulations, observations, and implications for nucleation mechanisms, J. Geophys. Res., 115, D17205, https://doi.org/10.1029/2009JD013473, 2010.
Yu, F., Luo, G., Liu, X., Easter, R. C., Ma, X., and Ghan, S. J.: Indirect radiative forcing by ion-mediated nucleation of aerosol, Atmos. Chem. Phys., 12, 11451–11463, https://doi.org/10.5194/acp-12-11451-2012, 2012.
Yu, F., Nadykto, A. B., Herb, J., Luo, G., Nazarenko, K. M., and Uvarova, L. A.: ternary ion-mediated nucleation (TIMN): kinetic-based model and comparison with CLOUD measurements, Atmos. Chem. Phys., 18, 17451–17474, https://doi.org/10.5194/acp-18-17451-2018, 2018.
Zhang, K., O'Donnell, D., Kazil, J., Stier, P., Kinne, S., Lohmann, U., Ferrachat, S., Croft, B., Quaas, J., Wan, H., Rast, S., and Feichter, J.: The global aerosol-climate model ECHAM-HAM, version 2: sensitivity to improvements in process representations, Atmos. Chem. Phys., 12, 8911–8949, https://doi.org/10.5194/acp-12-8911-2012, 2012.
Zhang, R., Suh, I., Zhao, J., Zhang, D., Fortner, E. C., Tie, X., Molina, L. T., and Molina, M. J.: Atmospheric new particle formation enhanced by organic acids, Science, 304, 1487–1490, 2004.
Zhang, R., Khalizov, A. F., Wang, L., Hu, M., and Wen, X.: Nucleation and growth of nanoparticles in the atmosphere, Chem. Rev., 112, 1957–2011, https://doi.org/10.1021/cr2001756, 2012.
Zhang, Y., McMurry, P. H., Yu, F., and Jacobson, M. Z.: A Comparative Study of Homogeneous Nucleation Parameterizations, Part I. Examination and Evaluation of the Formulations, J. Geophys. Res., 115, D20212, https://doi.org/10.1029/2010JD014150, 2010.
- Abstract
- Introduction
- Nucleation rate lookup tables for BHN, THN, BIMN, and TIMN
- Comparison of BHN, THN, BIMN, and TIMN rates from the lookup tables with CLOUD measurements
- Comparison of BHN, THN, BIMN, and TIMN rates based on the lookup tables with those based on other models and parameterizations
- Code and data availability
- Author contributions
- Competing interests
- Financial support
- Review statement
- References
- Abstract
- Introduction
- Nucleation rate lookup tables for BHN, THN, BIMN, and TIMN
- Comparison of BHN, THN, BIMN, and TIMN rates from the lookup tables with CLOUD measurements
- Comparison of BHN, THN, BIMN, and TIMN rates based on the lookup tables with those based on other models and parameterizations
- Code and data availability
- Author contributions
- Competing interests
- Financial support
- Review statement
- References